Creating Photodetectors from 2D Heterostructures
07-10-2020 | By Liam Critchley
Photodetectors are now used in a wide range of optoelectronic applications, from optical fibres to scientific characterisation equipment, and many more in between. They are mostly used in imaging and communication applications and are a device which captures photons of light and convert them into a detectable and readable electrical output.
Over the years, scientists have used a range of materials to build photodetectors and use materials which are efficient at absorbing wavelength/photons of light. The utilisation of photoactive materials has meant that the field of photodetectors has already reached a high level, hence their use in a wide range of technologies.
Nevertheless, there are still challenges for photodetectors to make them compatible and efficient for several technologies, and this includes balancing the many beneficial properties that you find with different photodetectors. For example, some have high photosensitivity and coverage across a wide range of the electromagnetic spectrum. Some have a linear response, others are flexible, while others are highly compatible with complementary metal-oxide-semiconductor (CMOS) technologies.
The challenge now, with the growth of high-performance technologies within everyday society and in scientific research, is to combine as many of these beneficial properties into photodetectors that are suitable for multiple applications. To do this, a lot of work is still going into creating new, and more advanced, photoactive materials to act as the photon sensing surface, and perovskite materials are one of the materials gathering much interest.
The Growth of Perovskite Photodetectors
Perovskite materials are inorganic materials which have a chemical structure of A2+B4+X32—where A and B are positively charged cations and X is a negatively charged anion. Even though perovskites show some excellent light absorption properties, they are inorganic materials, and they are rigid and inflexible. This had led to organic-inorganic perovskite hybrid materials being created which are much more flexible.
Hybrid perovskite photodetectors have shown to possess a lot of beneficial properties that make them a suitable material in a range of optoelectronic devices. Some of these benefits include a high light absorption coefficient, a low exciton binding energy, long electron-hole diffusion lengths, and cost-effective manufacturing methods (typically solution processing methods).
Despite the many beneficial properties, many perovskite photodetectors still have some key issues that need addressing. Many of these photodetectors tend to exhibit low photo-responsivities and low carrier mobilities and typically have low photoconductive gain mechanisms—meaning that they struggle to produce multiple charge carriers from a single photon (a process which enhances sensitivity as there are more detectable charge carriers that can be detected). So, new ways of using perovskite materials are being devised, one of which is to incorporate 2D material perovskite films in heterostructure formations.
2D Material Heterostructures
2D materials are nanomaterials which have a thickness to one to a few layers in thickness, so are inherently flexible. Despite the name suggesting that they are 2D in structural nature (i.e. one atom layer thickness), they can be a few atoms thick, because the ‘2D nature’ of these materials arises due to the quantum confinement of the charge carriers (i.e. the electrons) in 2 dimensions.
The inherent thinness of 2D materials means that they can be stacked on top of each other without chemically linking the layers (a bit like graphite has layers of graphene which are not chemically bound, but heterostructures always contain at least two different 2D materials—hence the term ‘hetero’ in the name).
The different layers are intermolecularly bonded, typically using van der Waals interactions (which is why 2D material heterostructures are typically called van der Waals heterostructures), so are physically held in place on top of each other without needing to link them chemically. In many cases, heterostructures can exhibit benefits that are more enhanced than the individual constituents that they are made of and are still flexible compared to bulkier materials.
Combining 2D Heterostructures with Perovskites for Efficient Photodetection
Thin-film perovskite materials, i.e. 2D materials made of perovskite structures, can be combined with other 2D materials to create heterostructures that are well-suited for photodetectors. Many perovskite heterostructure phototransistors have been developed over the years—using perovskite materials with graphene, molybdenum disulphide and black phosphorus—that can be used in photodetectors.
Many of these phototransistors work by separating the electrons and the holes at the heterojunction interface when it absorbs light. However, this mechanism means that the layers have to be doped, the charge separation can reduce the photogain of the device, and the migration of ions can cause hysteresis issues. So, while many of these phototransistors are efficient at absorbing light, there are some mechanistic aspects which are less desirable, so alternative ways of utilising perovskite heterostructures (mechanistically) are being sought.
A team of researchers from China and Hong Kong has now created an aluminium oxide/molybdenum disulphide (Al2O3/MoS2) heterostructure that has a different working mechanism to many other perovskite heterostructures. The research team devised this dielectric material to work via a charge trapping mechanism within the Aluminium oxide layer, and the hysteresis loop found in this arrangement is opposite to the ones seen in many other perovskite heterostructures.
This new mechanism helps to remove the hysteresis issues found in many other perovskite heterostructures because the charge trapping mechanism of the Al2O3 layer neutralises the ion migration at the heterojunction interface. The result is that this heterostructure not only possess a way to negate the hysteresis issues, it also has an enhanced photoconductive gain and an improved bias stress stability.
The photodetector can be modulated and does rely on the external biasing field to recombine the charge carriers. However, this also means that the photodetector is tuneable, so can be made highly efficient. One of the other key points is that this photodetector has ultrahigh photosensitivity because it can detect photons from the visible to the near-infrared regions of the electromagnetic spectrum.
Conclusion
So, while most 2D perovskite heterostructure phototransistors exhibit many of the beneficial properties required of modern-day technologies, they suffer from some drawbacks, particularly hysteresis issues. The recent advancement to use an Al2O3 perovskite layer with molybdenum disulphide has helped to negate these issues as well as induce high levels of photoresponsivity into the detector. These properties will be useful for a wide range of applications, and high-performance photodetectors could be made using such materials (provided they can be made at scale) for a wide range of optoelectronic devices and systems. They could potentially phase out some of the more traditional microelectronic photodetectors in use today.
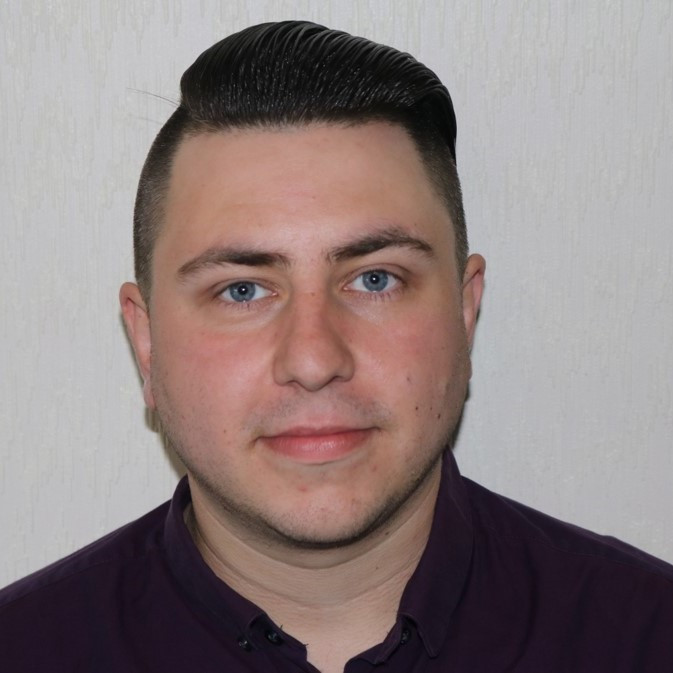