Creating Piezoelectric Generators from MXene-Fluoropolymer Composites
23-06-2021 | By Liam Critchley
As the interest in wearable and remote technologies grows, there is also a growing interest in ways to power these devices to be completely self-sufficient and not be reliant on a battery that will need changing. Many interest has been shown in nanogenerators harvesting energy from their surroundings, particularly in remote monitoring and medical applications where the electronics will need to be self-sufficient for long periods.
The small size of nanogenerators makes them suitable for many applications, and one specific type being investigated is piezoelectric nanogenerators. These types of nanogenerators use the piezoelectric effect to generate electricity. So, in effect, they take physical movements from their surroundings and convert these physical forces into an electrical charge that can then be used to power small devices.
Piezoelectric nanogenerators are some of the most common nanogenerators because there are several inorganic and solid-state materials that exhibit the piezoelectric effect. But there are also specialised organic materials emerging that can also exhibit the piezoelectric effect under certain conditions. One of these is fluoropolymers (and their composites). Regardless of the type of the material, the material itself needs to be capable of rearranging electrical charges at the molecular level. For some materials, this comes naturally, but for materials like fluoropolymers, they need to be polarised first, and there is currently a lot of research going into trying to make polymers piezoelectric as an alternative to solid-state materials.
Polarising Fluoropolymers
There are a number of different methods to induce polarisation into fluoropolymers so that they can exhibit a piezoelectric effect. For a polymeric material, that is typically dielectric (insulating) in nature, the best way is to align the dipoles within the polymer so that they can convert mechanical energy to electrical energy.
One of the more traditional methods of polarising these materials is to use electrical poling. However, this a very energy intensive process. Moreover, fluoropolymers are semi-crystalline materials, and the dipole moment sits between the fluorine and the hydrogen groups on the carbon chain, so an elevated temperature is also required to polarise them.
While polymers are a much cheaper option than many inorganic piezoelectric materials, the processing conditions required to make them suitable for energy harvesting devices makes them commercially unfeasible. So, newer ways of utilising fluoropolymers without needing a high energy input are being sought. One of the ways that has emerged is to use the polymers with different nanomaterials to create polymer nanocomposites.
Polarising Fluoropolymer Nanocomposites
Even though other wet chemical methods—such as tuning the solvent composition of the polymer—can help, one of the more promising routes to achieving low-energy polarising conditions is through nanomaterials. To date, templating the polarisation in these composites has been possible through a number of different nanomaterials, including barium titanate nanoparticles, reduced graphene oxide, hexagonal boron nitride and single-walled carbon nanotubes.
However, while the addition of different nanofillers has shown promising results, the mechanism of dipole alignment in these composites is poorly understood and if this area is to be commercially feasible, the fundamental mechanisms need to be understood alongside the benefits and application possibilities. This is mainly due to the nanomaterials trialled to date already possessing piezoelectric properties themselves, making it difficult to understand how the composites exhibit the piezoelectric effect.
Because of this, there has been interest in finding out the underlying dipole alignment templating mechanisms so that researchers can best choose the correct materials and tailor the properties of the energy harvesting materials to fit different applications. To probe these mechanisms, the nanofillers inside the composites need to possess out-of-plane polarisability without out-of-plane piezoelectric properties. Moreover, the nanomaterial needs to be rigid and have well-defined surface properties.
Taking all the different factors into account, it has emerged that MXene materials could be used to better probe how to polarise fluoropolymers and make functional devices. MXenes are two dimensional inorganic materials and consist of two or more layers where transition metal atoms sandwich layers of carbon or nitrogen between them. They are a growing class of nanomaterials so there is the potential to create a wide range of tailored devices.
The Potential to Create Creating Piezoelectric Generators from MXene-Fluoropolymer Composites
One of the most common and well-studied MXene materials is Ti3C2Tx, and because it has an out-of-plane polarisability, and its symmetrical structure lends itself to possessing out of plane piezoelectric properties, the researchers used this in their study. The researchers used a combination of physical experiments and molecular dynamics computations to achieve this.
The research showed the electrostatic interactions between the MXene nanosheet and the fluoropolymer play a key role in inducing local polarisation, as well as locking in the polarisation within the composite. There is a strong electrostatic interaction between both the polymer and the nanosheet, which results in the polarisation being ‘locked in’ perpendicular to basal plane of the MXene.
In electrical poling, the applied electrical field aligns the randomised dipoles in the fluoropolymers. Conversely, when the MXene is added to form a composite, the dipoles align without the need of an electric field, and when the solvent is removed, these polarisations lock in place with no relaxation. With electrical poling methods, there is also some relaxation of the polarisation, so this approach also provides a stronger polarisation as well as a lower energy route.
It’s not something that is observed with other materials, including other 2D materials. Knowing this, the researchers took this mechanistic understanding and was able to use extrusion printing or solvent casting to translate this localised polarisation into a macroscale polarisation throughout the composite, making it suitable for energy harvesting devices. Moreover, tuning the surface of the MXenes enables different electrostatic interactions to take place, meaning that these devices could be easily tuned to meet applications demands.
In terms of the piezoelectric nanogenerator created by the team, the device contained a fully polarised composite that had a piezoelectric coefficient of 52.0 pC N-1 and could harvest energy over 10,000 cycles without any performance degradation. Given that the polymeric materials are cheaper than other piezoelectric materials, the device shown could be both cost-effective and energy efficient and have the potential to be scalable because it is a low-energy production process.
Throughout this study, the research has achieved two overall objectives. The first is that they have manage to understand how MXene nanofillers without internal piezoelectricity effects can influence the macroscale piezoelectric properties of fluoropolymers. Following on from this, they were able to show how this fundamental understanding could be used to build fluoropolymer nanogenerators that don’t require high temperature and high energy processing stages.
The ability to produce a low-energy production route for these nanogenerators could help to unlock fluoropolymers for future nanogenerator applications, and this could be useful as the properties of these composites can be tailored to different applications much easier than inorganic, solid-state materials can. The developments here are not focused on one single device but are more about unlocking the potential of the whole device class (and being able to tailor them to demand), and developments such as these could help in creating bespoke devices for robotic interfaces, biomedical implants, and direct-on-organ printed electronics.
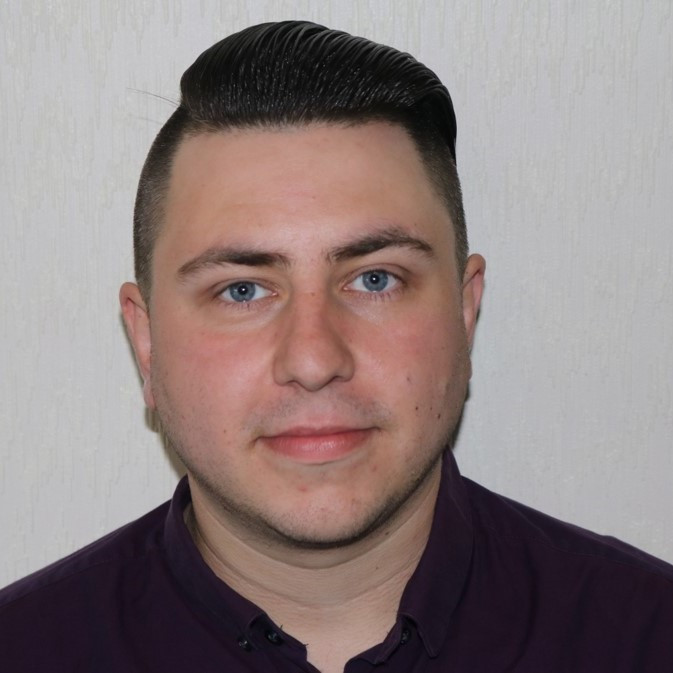