Researchers demonstrate scalable single-molecule sensors
02-02-2022 | By Robin Mitchell
Researchers have recently demonstrated a full-scale integrated circuit that can detect individual molecules. What challenges does individual molecular detection face, what did the researchers demonstrate, and where could this technology be used?
What challenges does molecular detection face?
When it comes to molecular science, the ingeniousness of researchers cannot go understated. Trying to understand how individual molecules fit together, the angle between different atoms, and how those molecules interact with each other when it is virtually impossible to see molecules is an incredible feat of human understanding. For example, researchers are convinced that water consists of one oxygen atom and two hydrogen atoms and that the angle between these atoms is 104.5˚. Still, no one has actually seen an individual water molecule, nor has anyone taken a protractor to the individual atoms.
The reason why molecules cannot be observed in the same way as we can observe everyday objects is due to the fact that, unlike large solid objects which reflect light, molecules are smaller than the wavelength of visible light, and this means that they cannot reflect light back in a way that would allow for an image (i.e. atom spheres stuck together). Of course, molecules can absorb and emit light, but light could never be used to resolve an image of a molecule (thus, a true image of an individual molecule would be a single speck of light).
Molecular detection can be done using spectroscopy, which looks at abortion and emission lines, but this only tells you the concentration of molecules as opposed to detection of individual molecules and could never be used to detect individual molecules. This technique also examines how molecules react (or what products are produced from a reaction) but does not tell you exactly how those molecules bind and react.
To summarise, detecting individual molecules is a massive challenge simply because we do not have detectors that can detect individual molecules. Instead, modern sensors rely on a minimum threshold quantity before they can detect the presence of a molecule, and even then, individual molecules in that solution cannot be differentiated and observed.
Researchers demonstrate the world’s first scalable electronic molecular detector
Recently, a team of researchers from Rice University have demonstrated the worlds first single molecular sensor that is scalable and practical in design. The new sensor not only allows for specific-molecule detection programming, but it can also do so in real-time. Furthermore, the sensor actually consists of a pixel array of 16,384 (16K) sensors which can be read in its entirety at a rate of 1,000 frames per second.
To create the molecular sensors, the researchers took two nanoelectrodes that are separated with a 20nm gap. This gap is then bridged using a molecular wire with a defined resistance, and applying a voltage across the gap results in a current flow (in the picoamps).
The molecular wire can then have a detector molecule attached, which makes the whole setup sensitive to a specific individual reaction between the detector molecule and another molecule. During a reaction between these two molecules, the resistance of the molecular wire changes, and this can be detected as a digital signal (reacted or unreacted).
The new sensor is especially powerful because it can be used with biochemical reactions and allow for the direct observation of enzymes and DNA. In the published paper, the researchers also discuss how their sensor can be incorporated with an enzyme designed to make copies of DNA so that a DNA strand can be read letter by letter.
Fig. 1. Molecular electronic sensor and chip concept. (A) Senor concept: Given a pair of molecules that undergo an interaction, one of the pair is selected as a probe molecule and conjugated to a precision molecular wire (here a synthetic α-helical protein) that spans a nanoscale gap between metal nanoelectrodes. These connect it to a driving voltage source and current monitoring circuit to provide real-time readout of current vs. time, for the current passing through the molecular wire/probe complex. When the target molecule binds to the probe, the resistance of the complex changes, resulting in an observed change in current. The resulting current trace has on/off pulses that provide a direct representation of the molecular interactions. (B) CMOS chip device: A large-scale array of sensors are fabricated on the surface of a CMOS chip. Shown is an annotated image of the CMOS chip device used in these studies. This chip has 16,000 sensors and the circuitry needed to digitize and transfer sensor readings off-chip, at a rate of 1,000 frames per second. (C) SEM image of sensor nanoelectrodes, showing the 20 nm gap for the molecular bridge. Nanoelectrodes shown are fabricated by photolithography, using CMOS foundry-compatible process.
What applications could such a molecular sensor be used for?
One application that stands out is rapid PCR tests. A Polymer Chain Reaction test involves taking a strand of DNA and then cloning the DNA strand until it is easily detectable with sensors, but this can take many hours of repeated cycles. If the new sensor developed by the researchers can be bound to enzymes used to clone DNA, they would be able to read out the entire sequence of a DNA strand without waiting for hours. This rapid speed would allow for faster detection of viruses such as COVID and could be a vital technology for pandemics in the future.
Another application for such a sensor would be in biosensing that could detect cancer in blood samples, toxic compounds in food and water, and even determine the state of health from sweat. If combined with AI, the new sensor could even provide predictive capabilities to health and identify molecules that could indicate dangerous health conditions long before current methods can.
In many cases, research papers and announcements on developments are little more than hot air with little practical capabilities, but what the researchers have demonstrated here is a fully working molecular sensor. All the researchers have to do now is turn their attention to developing detector molecules and seeing how these could be used in preventative medicine.
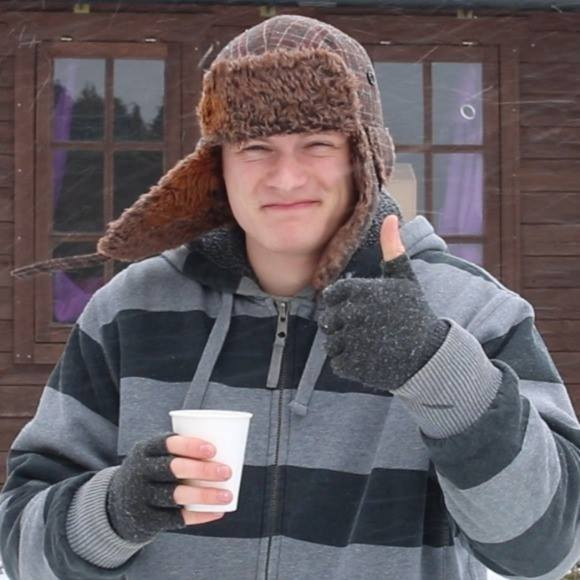