Could We Use Bacteria to Build Bioelectronic Systems?
| 07-03-2022 | By Liam Critchley
The areas of synthetic biology and biomaterials are ever advancing, and new ways of utilising the natural world continue to manifest in modern-day scientific research. There has been a drive to harness the natural world and naturally occurring biomolecules to create electronic systems in recent years. This is the growing area of bioelectronics.
There have often been limits to the efficiencies that can be created by humans with synthetic materials. However, the natural world has created chemical and pseudo-bioelectronic systems far more advanced than we currently produce. So, by trying to harness these naturally occurring biomaterials, scientists are also hoping to emulate nature’s success.
One area of the natural world that is of particular interest is the ability for biological systems to utilise sequence-controlled processes for synthesising different biomolecules, where the molecular information is encoded within nucleic acids and subsequently converted into sequence-controlled polymers. These processes are of interest because they can be used to create natural protein-polymer materials that are potentially suitable for different electronic devices. However, while protein polymers have special functions in nature, with a range of beneficial mechanical and structural properties, they do lack functional electronic and optical properties, which inhibit their use in bioelectronic applications.
These natural polymer materials can, however, be engineered to be more functional from an electronic perspective. The natural properties—ranging from structural stability to the ability to regenerate, sense, and adapt to their environment—make them attractive candidates if they can be improved electronically. One of the key constraints of conventional biomolecules is that there are only 20 suitable amino acid building blocks for these proteins. One of the ways to potentially expand the scope of bioelectronics is to expand beyond the conventional proteins that we use from plants and look at other aspects of our natural world—namely, bacterium.
The Potential for Bacterial Pili
If scientists are going to go down the route of utilising natural polymer proteins from bacterial sources, then bacterial pili could well be the answer. Pili are filamentous proteins that grow on the surface of bacteria and play a key role in how bacteria interact with our bodies—primarily via acting as a biosensor. These pili filaments have a lot of potential because they have a high mechanical robustness and self-assembly ability while being able to withstand a wide range of temperatures and pH’s. However, like many of the high aspect ratio protein filaments, they lack electronic and optical properties. Nevertheless, the other properties are beneficial for bioelectronic applications, and there is scope to make these filaments more electrically conductive and more suitable for bioelectronic devices.
Making Bacterial Pili Conductive for Bioelectronics
Electrical conductivity in bacterial pili has been found across its diameter but not across its length. If conductivity can be implemented along its length, these pili could be used as biological nanowires in bioelectronic devices. Beyond this, another challenge is the agglomeration of these pili, but if they are to be functional in a device, they need to be appropriately aligned to allow electricity to flow efficiently.
Recent developments in this space have looked at how bacterial pili can be made conductive and made suitable as nanowires in bioelectronic systems. Researchers have now managed to engineer a high level of electrical conductivity into pili from E. coli. This was achieved in three stages. Firstly, amino acid (tryptophan) mutations were encoded into the pili, which allowed an 84-fold increase in the conductivity of the filaments compared to their natural state. These conductivity figures were also recorded for each individual filament rather than filament films, showing that individual filaments can act as nanowire building blocks if manipulated correctly.
The second stage of research involved engineering long-range conductivity over the micrometre scale. This was achieved by creating hierarchical nanostructured networks using self-assembly methods. This approach enabled the nanostructured network to achieve a five times greater conductivity than unordered pili networks. The final approach was to encode the pili to form a click-chemistry-functional scaffold that allows gold nanoparticles to be integrated into the pili network. This final approach further increased the electrical conductivity of the individual filaments to achieve conductivity values that are 170 times greater than the pilis natural electronic state.
So, the big question is, would it be possible to use bacteria to build bioelectronic systems. At a fundamental level, it’s definitely possible, as recent developments have not only created highly conductive protein filaments that can behave like nanowires, but they have also managed to keep them ordered so that they can deliver an electrical current effectively. All in all, it’s an interesting system that has been chosen to create conductive biological nanowires. So, it could be entirely possible to use these conductive filaments as nanowires in different bioelectronic devices, and some of the applications being touted include sensors, transistors, and capacitors.
Now, looking to a larger industry and real-world level, that remains to be seen. While using bacteria as the ‘raw biomaterial’ helps expand the scope of available materials for bioelectronic devices, the big question is how scalable these technologies are going to be. —especially when you need to isolate these proteins followed by several encoding/synthesis steps before getting the desired product. Other biomolecules are more readily available, but it doesn’t mean that they are necessarily suitable. A lot of it is likely to come down to how the electronics industry values the potential for bioelectronic devices in the future compared to how much it is seen as an ‘interesting’ research area.
In any case, given the scope of modern-day electronics, if bioelectronic systems are going to be used commercially, they’re probably going to have to find their own application/market sector niche (e.g., certain biological/medical applications) where they are not trying to compete with existing synthetic technologies (and the rapid expansion of advanced synthetic materials in the technology space), but it is still a relatively new area when you compare it to the existing and longstanding synthetic electronic industry.
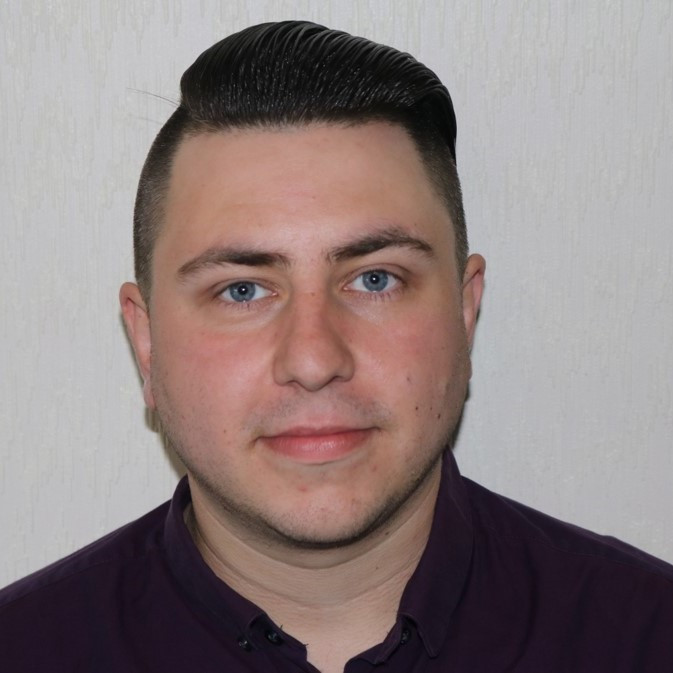