Putting the Focus on SEI Engineering in Solid-State Batteries
Insights | 07-12-2022 | By Robin Mitchell
Li-ion batteries are still the dominant battery technology, but there is a range of other lithium-based solid-state batteries that show a lot of promise, especially for electric vehicles (EVs). Theoretically, it’s possible to create lithium metal anodes with theoretical capacities of 3860 mAh g-1 that can lead to battery cells with gravimetric and volumetric energies greater than 400 Wh kg-1 and 1000 Wh L-1, respectively.
However, there’s a reason that Li-ion is the dominant battery technology today, and it’s because other lithium-metal batteries have a number of challenges that have made them less commercially feasible. Lithium has a strong reduction potential, and it often reacts with solid electrolyte materials, resulting in a solid electrolyte interphase (SEI) that is both kinetically and thermodynamically unstable.
The presence of these interphases results in performance degradation and cell failure because they facilitate a poor electrolyte-electrode contact, as well as inhomogeneous plating/stripping and the formation of voids and lithium filaments. All these factors have contributed to less-than-ideal battery performances and stunted the commercial potential of many Li-metal batteries.
Understanding the chemical composition and morphological evolution of the SEI formed between the lithium metal electrode and the solid-state electrolyte is going to be crucial going forward if reliable and commercially feasible all-solid-state lithium batteries are to be realised. Researchers have now probed the SEI layer of lithium solid-state batteries using out X-ray photoemission spectroscopy (XPS) to see how the current affects the SEI layer and how this can be engineered to achieve a more optimal and stable performance for Li-metal batteries.
SEI Formation in Li-Metal Batteries
The SEI is a thin layer that forms during the first discharge of a battery when the electrolyte reacts with the electrode. This reaction occurs when the redox potential of the electrodes lies outside the electrochemical window of the electrolyte and can either have a detrimental or positive impact on the battery (such as for preventing the degradation of the electrolyte) depending on the dynamics of the cell and whether the SEI layer is stable or not. So, finding the right electrolyte for the electrodes used is key and is why many Li-metal batteries have struggled to gain commercial traction.
Li6PS5Cl (LPSCl) is a solid-state electrolyte that has emerged as a potential option. LPSCl does have a limited electrochemical stability window, but it is already known to produce a kinetically stable SEI when in contact with metallic lithium. This stable SEI layer is known to prevent further degradation of the electrolyte and is a beneficial SEI layer, but it does have a negative impact on the electrochemical performance of the battery because it increases the interfacial impedance.
When it comes to LPSCl, the degradation products are LiCl, LixP and Li2S (the latter of which is partly responsible for degradation in Li-S batteries). All of these are poor Li+ ion conductors, and the formation of these molecular products directly affects the cycling capacity and coulombic efficiency of the cell.
SEI Mechanism Formation in LPSCl
Using thermodynamic calculations, it’s been theorised that LPSCl decomposes in the cell via a multi-step mechanism that loosely goes from LPSCl to Li2S, phosphorus and LiCl intermediaries before further decomposing to Li3P. The transition occurs through partially reduced phosphide molecules which form in Li-deficient conditions. While some of the intermediary species are poorly conductive, the fully reduced Li3P has good ionic conductivity and a good stability when in contact with lithium metal.
These insights and understanding of how the interface degrades offer an approachable way for battery researchers to better engineer the interfaces so that they remain stable at high currents. Efforts to improve the stability at high currents have taken the form of anode interlayers, Li alloys and high stack pressures, but all of these have had a negative effect on either the energy density or the fabrication potential at commercial scales.
An approach has come to the fore in recent times where electrochemical pulses are used to decrease the interfacial impedance between the lithium metal and oxide-based solid electrolytes. Results show that this approach forms both kinetically and thermodynamically stable interphases because of an improved lithium-electrolyte contact. Even though it’s known that the interfacial reduction process is kinetically driven, there is some unknown information around the evolution of the SEI itself and how different currents affect the SEI layer.
The Effect that the Current has on SEI Formation
Researchers in the UK have now looked further into LPSCl and how the SEI layer can be altered by changing the current across the cell. To do this, the researchers used XPS to investigate the current density-mediated evolution of the interphase formed between the lithium metal and LPSCl during lithium plating.
The researchers chose a ‘lithium-free’ negative electrode configuration to investigate the formation and evolution of the SEI layer because the negative electrode was devoid of any metallic lithium at the time of assembly—making it more accurate for determining how SEI formation kinetics and composition have an effect on the lithium-electrolyte electrical impedance.
The approach taken using XPS is also known as ‘virtual electrode plating’ because the approach forms a ‘virtual electrode’ when the grounded and Li-backed solid electrolyte surface is exposed to the electron beam. The negatively charged surface that gets formed on the electrolyte by the electron beam facilitates the migration of Li+ ions, leading to the plating of metallic lithium on the surface of the solid electrolyte.
In this research, the electron beam current was also adjusted to modulate the electron flux at the surface of the solid electrolyte. This was the main way of tuning the virtual electrode plating current, and the electrochemical response was observed via the evolution of the interface. This approach was finally adapted in the research on a full solid electrolyte cell to study the plating of lithium on a stainless steel collector at different current densities.
At high current densities, during the initial plating stage, the interphase was found to be rich in Li3P molecules, showing that the decomposition product was fully reduced. It was also found to be a uniform layer as well. The higher current density effectively led to a more conductive SEI layer that has a lower interfacial resistance. The results were confirmed by measuring the spectroscopic signal of the surface with XPS and further confirmed with electrochemical impedance spectroscopy (EIS). The research suggests that reaction kinetics plays a major role in the plating process.
Because it was found that higher current densities resulted in a more conductive and uniform SEI layer, this approach and subsequent findings could be utilised in future studies for engineering the electrode-electrolyte interface and develop charge-discharge protocols that can improve the lithium plating properties in solid-state batteries. With the drive for developing batteries beyond Li-ion and the challenges that have faced other battery technologies, new ways of engineering a better performance are always a welcome addition to the research landscape.
Reference:
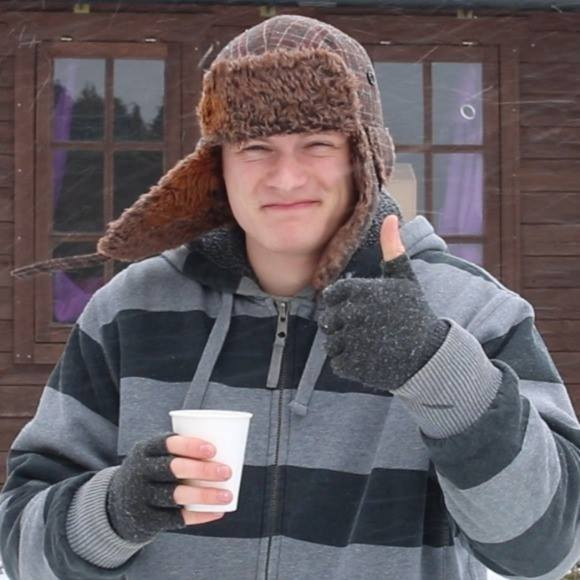