Creating Terahertz Waves with A Thin-film Lithium Niobate IC Platform
31-01-2023 | By Liam Critchley
The terahertz (THz) region (0.1-10 THz) of the electromagnetic spectrum is crucial for a number of modern-day technologies, including in communications, sensing, medical imaging and quantum technologies. THz radiation waves are used throughout the sciences and play a fundamental role in spectroscopy-based analyses because the THz range hosts low-energy material resonances, and these spectroscopy analyses are now playing a pivotal role in many areas, from security to medical diagnostics.
THz waves are of interest in these application areas because they are non-invasive and non-ionizing. They are very useful for non-invasive medical imaging procedures as they offer a higher degree of resolution for distinguishing between different materials than is possible with microwaves. But the utilisation of THz waves goes even further and can be used to control excitations at the fundamental atomic level, including controlling electron spins, molecular motion, and photons faster than microwaves, and this is where the fundamental process has become useful for communication, sensing and quantum applications.
For all the applications, the speed of THz waves over other wave-technologies (like microwaves) is one of their main driving factors for use. Of all the applications, communications have the largest potential impact because communication systems continue to get faster and faster, and there is an ever-growing need for faster and more efficient communication systems.
THz waves offer the possibility to use narrow-angled directional beams as an information channel rather than the wide-angled broadcasting beams seen in traditional microwave communication technologies. However, bridging the gap from a wider communication waveform to a narrow waveform relies on creating custom waveforms that are tailored for each application. However, this is typically difficult to do with bulk crystal materials as they have limited flexibility for optical rectification of femtosecond pulses, so scientists are now looking towards thin-film platforms for the answer.
Controlling THz Waves
The large-scale realisation of THz communications will hinge on the ability to control the THZ fields at sub-THz scales, so having a way to synthesize these waves at will and in a controlled manner, is seen as of vital importance. As far as control goes, the customisation of the waves requires their amplitude, frequency, and phase down to a single cycle of oscillation to be specifically tailored to the application at hand.
As it stands, the THz range is challenging to access via optical and electronic technologies, and while a good amount of progress has been made towards the miniaturisation of some THz devices, their emissions tend to be in the upper THz domain, or there is limited control over the frequency of the output wave. Of all the optical components, one of the more promising methods for generating and detecting THz waves is optical rectification devices using a near-infrared (NIR) laser. In terms of the materials available for creating and controlling THz waves, lithium niobate shows a lot of promise because it has low optical losses in the NIR region.
While optical rectification shows promise, generating THz waves has only really been feasible so far using bulk materials. Bulk crystals are well-suited for creating tailored THz waves through several mechanisms, but there are a number of issues with their use. These include the need to pre-condition the laser or post-condition the pulse to make it a tailored wave, an uncontrollable refractive index mismatch (resulting in phase delays) at THz and pumping frequencies, the need to pump the laser close to the rectifier to avoid the THz radiation being absorbed and achieving sub-THz-cycle precision in the waveform is challenging. There’s been a lot of work going into the area, and creating thin films of lithium niobate could offer a way to overcome these challenges thanks to advances in nanofabrication and nanoengineering methods.
Creating a Thin-Film Platform for THz Wave Formation
Researchers have now looked at creating thin-film lithium niobate integrated circuits (ICs) to combat the challenges associated with THz generation in bulk lithium niobate crystals. By combining complex integrated architectures, low-loss distribution of pump pulses on-chip, and an efficient optical rectification, the researchers have now been able to develop a platform that can generate a versatile range of THZ waves.
Using low-loss integrated photonic circuits, they found that they could avoid the challenges of bulk THz wave generation systems and exert a greater degree of control over the temporal, spectral, phase, amplitude, and far-field characteristics of the generated waveform. This is thanks to a distributed pulse phase-matching scheme brought about by the designed on-chip components. This phase-matching scheme not only provides the platform with a way of avoiding the phase-delay mismatch seen in bulk crystals, but it also removes the need for any pre-conditioning/pre-engineering to be done to the laser pump beforehand.
The platform’s success is thanks to nanoengineering methods that wouldn’t otherwise be possible/workable in bulk crystals. For example, rib waveguides were employed on the IC to allow THz generation within a few hundred nm of the surface, which in turn minimised the amount of THz absorption by the lithium niobate.
Additionally, because the thin film lithium niobate is a low optical loss material, it enabled several basic components―including grating couplers, y-splitters, and combiners―to all be put on a single chip and be used together to create the THz waveform. When the ability to pack all these components onto the chip was combined with the wide transmission window and high-power resilience of lithium niobate, the laser pump pulses were guided at energies of 100 pJ through complex device architectures without any major loss or material breakdown.
Another aspect is that the monolithic patterning and design of THz antennas around the waveguides offered a way of controlling the spectral and temporal properties of the emitted THz radiation, as well as controlling its emission into the far-field. The final engineering success is that the approach enabled the waveguide dimensions to be chosen, which in turn provided a precise control over the mode index, the group index, and the field confinement of both the pump pulses and the THz field as they interacted with the antenna.
The choice of switching to thin films of an existing material that shows promise has enabled the researcher to construct a photonic IC device that can be used as a basic building block for creating THz waves―with broadband emissions up to 680 GHz and far-field amplitudes of a few V m-1. The approach has managed to overcome some of the key challenges of THz generation and control seen with bulk crystals, and researchers are currently targeting a wide range of applications for these thin film devices, including metrology, spectroscopy, quantum technology and communication applications.
Reference:
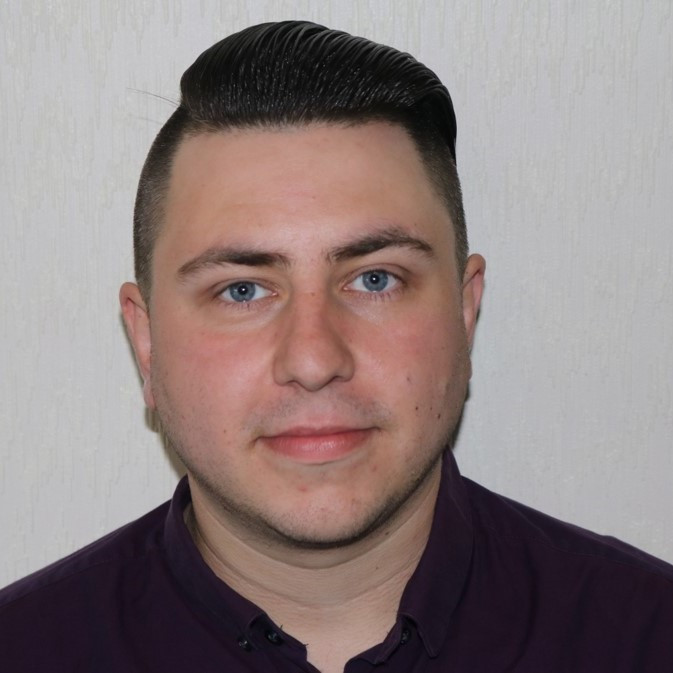