Is the Meminductor Real? New Circuit Element Discovered by Scientists
Insights | 20-04-2023 | By Robin Mitchell
Recently, researchers claimed to have made a new circuit element concept for a two-terminal meminductor whose inductance depends on the past current that flowed through the inductor. While their device does exhibit this behaviour, it is far from being practical in any real application but certainly provides exciting evidence for this behaviour. What exactly is a meminductor, what did the researchers develop, and does this research suggest that a real meminductor could exist?
What exactly is a meminductor?
For the longest time, scientists and engineers were convinced that only three fundamental circuit element existed; resistors, inductors, and capacitors. From these components, all other devices could be constructed, and they were mostly right about this. Incandescent bulbs are just resistors getting very hot in an inert gas, electromagnets are very powerful inductors, and energy banks can be constructed from an array of capacitors.
Of course, new materials were discovered that had unique properties, such as semiconductors. However, the difference between the three basic components and others, such as semiconductors, is that the three properties of resistance, capacitance, and inductance are entirely independent of each other. This means that one can be adjusted without affecting the other properties.
However, during the 1970s, scientists discovered the possibility of another three fundamental components whose value depends on past current flow. These components, called mems, have been mostly theoretical until researchers in the mid-2000s demonstrated components with memresistive properties.
The discovery of meminductance could potentially reshape the electronics industry. These unique components can store information about past current flows, which may lead to more efficient and compact devices. Applications could span from signal processing to advanced computing, such as adaptive filters with self-tuning abilities and neuromorphic circuits that mimic brain functionality. Despite the challenges in realising meminductors, their potential applications could have a significant impact on the future of electronic devices and systems.
But one component that hasn’t been physically demonstrated is the meminductor, a component whose inductance depends on the past current flow. It is hard to describe the behaviour of a meminductor due to the fact that the inductance change depends on the previous current flow and the direction it travelled in. For example, if the current in a memristor is reduced, the inductance that the meminductor has is different to the inductance when the same current flowed previously (forming a figure of 8 in a L/I chart).
When an AC current is passed through the coil, the coil moves along the tube towards the iron core. However, the interaction between the coil, iron core, and magnets changes the coil's inductance. The resulting plot of the current and inductance resembles the figure of 8 that would be expected in a meminductor.
Researchers create proof-of-concept meminductor
Recently, researchers from Texas A&M published a paper claiming to have created a two-terminal concept meminductor. In their paper, they describe the method of creating the meminductor using a tube half-filled with an iron core on one side, two magnets placed on either side of the tube with the same pole facing inwards, and a coil that can travel freely along the tube.
This breakthrough adds to the ongoing advancements in electronics and technology, as it showcases the first physical evidence of meminductance. The proof-of-concept meminductor brings us closer to understanding the potential applications of this new fundamental circuit element, which could revolutionise areas like energy storage and signal processing. By shedding light on meminductor behaviour, this research fuels the pursuit of cutting-edge electronic devices and systems that could shape the future of the industry.
Experimental Setup & Results: (a-d) Two neodymium magnets connected by a smooth shaft with like poles facing each other; the core partially filled by a ferromagnetic rod. Alternating current half-cycles (a, b) create alternating magnetic poles on the winding, resulting in periodic motion. Magnetic field patterns in (c, d). (e) Quasi-static inductance measurements on W-1 based on winding position. (f, g) Total voltage measured across winding terminals. (h, i) Total flux calculated. (j, k) Extracted (mem)inductive voltage. (l, m) Extracted (mem)inductive flux and instantaneous dynamic inductance. Top-right inset: experimental setup. (Click to enlarge)
Lead Researcher Comments on the Future of Meminductors and Challenges Ahead
Abhiram Dinavahi, the lead researcher, commented on their findings and the future direction of their work in the paper's conclusion, stating, "The element realised in this work has been shown to bear the three fingerprints of a meminductor and thus prove the physical evidence of meminductance, albeit overshadowed by a more dominant resistive component. Operating the element in a cryogenic environment below the superconducting temperature of the winding appears the most feasible technique to eliminate series resistance in the configuration discussed." This highlights the importance of their discovery while acknowledging the challenges that lie ahead in the pursuit of a practical meminductor.
The researchers are now looking towards material sciences to try and find materials that could realise a true two-terminal meminductor. It is believed that the first meminductors will be found at extremely low temperatures, where more unusual phenomena, such as superconducting properties and quantum effects, take place. For those who want to see the experiment on video, see the YouTube Short video below.
Exploring the Meminductor's Position on Chua's Periodic Table
Exploring the Meminductor's Position on Chua's Periodic Table An interesting aspect of this discovery is how it relates to Chua's periodic table of two-terminal circuit elements. The behaviour of a circuit element displaying a pinched hysteresis curve in the (v(α+1) − i(β+1)) plane identifies the element, allowing it to be grouped into different families.
In the case of the researchers' proof-of-concept meminductor, it exhibits pinched hysteresis in the (v(−1) − i(0)) plane, indicating it belongs to the inductor family. Furthermore, it demonstrates a deviation from ideal behaviour due to a delay in the change of the sourcing function and transfer function. This potentially renders the ideal memristor unfeasible while also placing the realised meminductor within the realm of generic meminductors due to its multi-valued response in the (v(−2) − i(−1)) plane.
Understanding the meminductor's position on Chua's periodic table provides valuable insights into its behaviour and properties, helping researchers identify future directions and potential applications for this newly realised circuit element. By examining the relationships between the meminductor and other known circuit elements, we gain a deeper understanding of the underlying principles that govern the fascinating world of electronics.
The device's position on the periodic table of two-terminal circuit elements can be seen in Leon Chua's periodic table, with the resistor, capacitor, and inductor families highlighted (a). The device exhibits meminductive properties as a result of the pinched hysteresis response in the (v(−1) − i(0)) plane (b) and is identified as a generic meminductor due to its multi-valued response in the (v(−2) − i(−1)) plane (c).
Does this research suggest that a real meminductor could exist?
The device developed by the researchers demonstrates how a meminductor could operate, but it is far from a practical device. A real and practical meminductor would likely be solid-state and capable of operating at much higher frequencies. However, future meminductors might be MEMS devices, using miniaturised coils, magnets, and structures.
The practical use for such devices is still yet to be determined, but their memory property could be beneficial for low-energy, long-term memory storage that doesn’t degrade with use. Such
components could also be used for creating new circuit configurations whose properties change over time (memristors, for example, have potential in neural nets, representing links between neurons).
Overall, what the researchers demonstrated here is very exciting, and while this device won’t be finding its way into everyday electronics anytime soon, future developments could be!
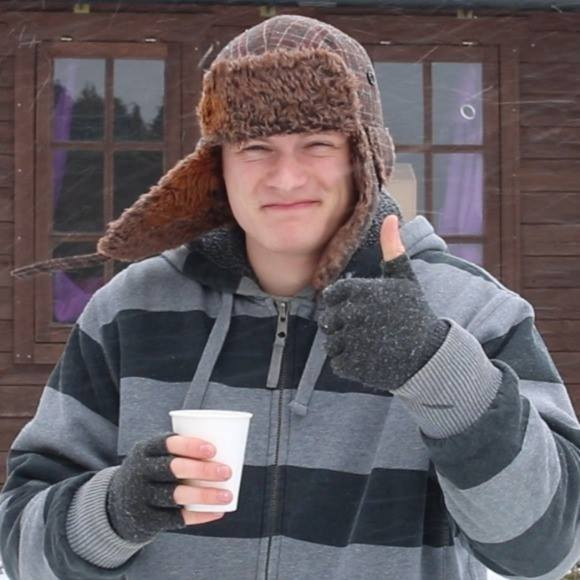