How Soft Interlayer Design is Transforming Stretchable Electronics
25-09-2023 | By Liam Critchley
Soft and stretchable electronic devices have gathered a lot of interest in recent years, primarily as tools for biomedical diagnoses and in the study of biological systems. This is because these devices can interface effectively with the human body and other biological systems. Many of these devices have been utilised as either wearable or implantable medical devices (IMDs), and their specific applications have ranged from health monitoring to treating diseases and performing fundamental biological research studies.
However, there has been a lot of focus in recent years on creating the stretchy materials required to conform to the wearer, but not a lot of research has been dedicated to the fact that a lot of these devices have a mechanical mismatch to the surrounding tissue. While materials have been made to be super stretchy and conformable (and there’s been some great work in this area), many of the devices have a Young’s modulus that is orders of magnitude higher than the soft biological tissues they are interfacing with.
A detailed view of a ribbon cable featuring copper lines in green and yellow, situated on a flexible PCB, commonly found in headphones.
Taking the Research Focus off Stretchability
The ability to interface electronic devices with the human body—in both a wearable and implantable capacity—is opening the door to many biomedical applications. While the focus has been on creating stretchy materials that can make many monitoring applications a reality, the focus now needs to be shifted from purely looking at the stretchability and tackling the mechanical mismatch that exists between these devices and the surrounding biological material.
There are a number of considerations that need to be taken into account—especially for implantable devices. The trade-off between the Young’s Modulus and stretchability is important for creating materials that have similar mechanical properties as the biological tissues around them. Also, suppose implantable devices have a similar softness as the surrounding tissues. In that case, it reduces the foreign body response invoked by the body when there’s a foreign object present—so this can also help to improve the overall biocompatibility of the device as the body is less likely to see it as a foreign object and attack it if its properties are similar to surrounding tissue. All these parameters lead to better conformability and an interface that is likely to be scar-free, and for biological monitoring applications, these aspects are key for signal transduction and modulation.
Achieving a modulus that is the same as the surrounding tissue requires ultrasoft materials. However, many of the materials used in a sensing capacity (i.e., the materials that are the active sensing surface in these devices) are conductors and semiconductors that have a much higher Young’s modulus, and their stretchability deteriorates when placed on substrates with a much lower Young’s modulus.
Large differences in Young’s moduli across interfaces can increase the stress conditions at defect sites and can cause a greater degree of crack propagation. To address the mechanical mismatch between the moduli on either side of the interface, research has turned towards using intrinsically stretchable conductor materials based on either hydrogel and aerogel networks or conductive nanomaterial networks that are dispersed inside matrices with an ultra-low Young’s modulus.
While a lot of progress has been made in the area, it has only been for passive electrodes and passive sensors for signal recording applications. A Young’s modulus that is the same as the surrounding tissue has not been created for the semiconductors that are used in more active devices—i.e., devices that control the charge flow— such as transistors, photodetectors, LEDs, and signal processing circuits. Researchers have aimed to change and further develop more active devices that are more compatible for long-term use at the tissue interface.
The recent study from Nature Communications delves deeper into the challenges of achieving tissue-level softness in stretchable electronics. The research highlights the importance of a soft interlayer design, which can significantly enhance the device's mechanical properties, making it more compatible with biological tissues.
A Soft Interlayer Design Creates a Compatible Device
Researchers have now developed a device that does not suffer from the conventional mechanical mismatch of other devices. This has been done using existing stretchable materials—that have a relatively high Young’s modulus—by using these materials to create a soft interlayer structure. While the materials are known to traditionally have a high Young’s modulus, this design approach enabled the creation of ultra-soft and stretchable devices with these materials—such as transistors and sensors—that have a modulus that is comparable with the ultra-low moduli of biological tissue.
Fig. 1: a. Overview of Young’s modulus for existing electronics, ultrasoft polymers, hydrogels, and typical bio-tissues. b. Diagram illustrating reduced stretchability due to stress concentration when an electronic film is stretched on a much softer substrate. c. Soft-interlayer approach using a medium-modulus film to minimise modulus disparity, enhancing stretchability of the functional layer. d. Young’s modulus comparison of an ultra-flexible transistor array on a PAAm hydrogel substrate versus conventional elastomers. Inset: Strain-stress curves of the transistor array and hydrogel. e. Display of the softer nature of the transistor array on PAAm hydrogel versus a design on an SEBS substrate under identical force. f. Conformable attachment of a soft transistor array on a finger joint in varied bends (top row), showcasing notable advancement over a standard device on an SEBS substrate (bottom row). Scale: 10 mm. (Click to enlarge)
It was found that creating layers that adhere to each other in an interlayer structure can improve the stretchability of an electronically functional thin film by over 100 times. The researchers particularly found that the polymeric material polystyrene-ethylene-butylene-styrene (SEBS)—with a Young’s modulus of 2.83 MPa—can be used as an ideal interlayer material and can be used with ultra-low modulus substrates such as soft silicones and hydrogels.
Innovative Applications of the Soft Interlayer Design
The researchers deposited a 1.2 μm-thick SEBS interlayer on a 200 μm-thick polyacrylamide (PAAm) hydrogel, which caused a negligible increase in the modulus of the device, and the overall platform had a modulus that is two to three orders of magnitude lower than commonly used substrates—including pure SEBS substrates and polydimethylsiloxane (PDMS).
The ability to deposit an active component onto low modulus substrate presented an opportunity for the researchers to improve on the current designs and set out to further these designs by creating a stretchable transistor array. These transistor arrays were built on a PAAm hydrogel with a Young’s modulus of 5.2 kPa.
Benefits and Potential of the New Design
This tissue-like softness of these interlayers (from the low kPa modulus compared to the MPa range of most other devices) means that these materials can be used to create more conformable and stable devices that can form better contact with biological tissues. The interlayer design increases the stretchability of the device by achieving a uniform strain distribution.
These transistor-based devices have been able to conform to irregular and dynamic surfaces and were subsequently tested to record electrophysiological signals on an isolated heart without influencing the pressure inside the heart once interfaced, as an on-skin device with low irritation, and as a subcutaneous implant. In-vivo tests have shown that the conformability and low modulus suppressed the immune response from the body, leading to a higher biocompatibility.
The ability to integrate stretchable semiconductors into ultra-soft substrates without affecting the stretchability of the device offers an efficient and versatile platform for creating a number of implantable and wearable medical devices with enhanced longevity. While this research has focused on integrating transistors into the device for sensing and health monitoring applications, this interlayer design could be further expanded for a range of other stretchable devices, including photodetectors, LEDs, energy-harvesting devices, and various other biosensors.
Furthermore, the study emphasises the potential of these devices in various biomedical applications. Their adaptability and biocompatibility make them ideal candidates for future innovations in the field of medical electronics, paving the way for more advanced and patient-friendly solutions.
Reference:
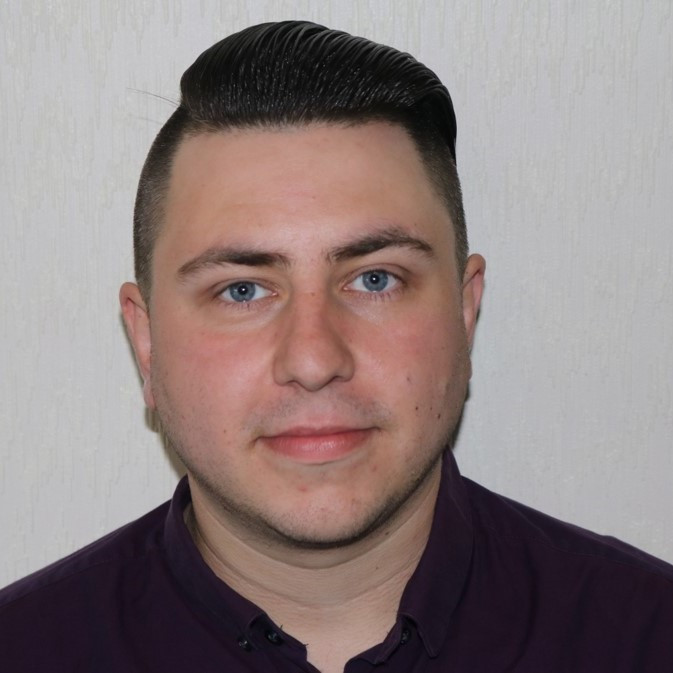