Curtin University's Breakthrough in Single-Molecule Detection
23-10-2023 | By Robin Mitchell
Recently, researchers from Curtin University (Australia) have developed a new single-molecule sensor that could potentially revolutionise the field of sensing. What challenges do sensors face when being shrunk in size, what did the researchers demonstrate, and how could it be revolutionary in future sensing applications?
What challenges do sensors face when being reduced in size?
Arguably, one of the most important features of electronic circuits is their ability to interact with their surrounding environment. In fact, even devices such as computers, which would typically be seen as not needing to sense their surroundings, must be able to detect their own temperature so they can prevent permanent damage from overheating.
Just like with all electronic components and devices, there is a strong demand for engineers to try and reduce the size of sensors as much as possible. Smaller sensors use up less PCB space, thereby allowing for the design to be reduced in size, have less mass, which reduces the overall weight of a design, typically use less energy and thus, increase battery life, and can allow for more complex on-chip functions (such as local AI in sensors).
But it is not just consumer electronics that see demand for smaller sensors; just about all applications that involve some kind of sensor demand smaller and more efficient sensors. This includes military, medical, industrial, and automotive sensors, and many of these industries will pay top dollar for miniaturised designs.
However, unlike transistors, which are fairly straightforward to reduce in size, sensors are not as simple as one would hope. Sensors based purely on the electrical properties of materials (such as thermocouples) can be shrunk with ease, but those that are based on mechanical principles can struggle significantly.
For example, sensors that use microelectromechanical parts (MEMS) utilise cogs, wheels, levers, and springs. While these can be reduced in size, their performance does not scale in the same way, especially for parts that rely on masses attached to springs. As such, trying to make them smaller can induce all kinds of headaches that engineers have to figure out.
Another challenge of making sensors smaller is that their reduced surface area can make them less sensitive. For example, chemical sensors that detect vapour require a large surface area for vapour molecules to make contact with the sensor. If that surface area is reduced in size, the number of reactions between molecules and the sensor is reduced, thereby reducing the electrical activity of the sensor.
Finally, sensors that are used to detect changes on the molecular level can struggle with noise and other atomic influences. Worse, trying to detect changes in individual molecular sensors in real time can be extremely challenging, especially if the sensor involves fluids that need to mix before being able to detect changes.
Researchers create single-molecule piezo device
Recognising the challenges faced with single-molecule sensors, a team of researchers from Curtin University (Australia) have recently published their work on a single-molecule sensor that they claim could very well shape the future of nanomolecular sensors.
Figure 1 illustrates alterations in molecular and interfacial structures both in solution and within NEMS devices.
a) In solution, bullvalene's fluxional nature is attributed to the Sigmatropic Cope rearrangements. b) When substituted with Diaryl (Ar = para (C6H4)‒SCH3), bullvalenes present as bent isomers during STMBJ experiments with minimal tip-tip distances. c) Specific tip extensions reveal bullvalene isomers with varying conductances in equilibrium, showcasing oscillating single-molecule reactions observable on a millisecond scale. d) Conductance is influenced by bullvalene isomerisation during tip retraction, showcasing piezoresistance. e) At reduced tip distances, bullvalene isomerisation prompts tip reconstruction. The blue hue in (b‒e) indicates potential electron routes.
The device, a piezo resistor, consists of a single diaryl-bullvalene molecule, a cage-like structure with the chemical formula C10H10. The researchers were able to manipulate the molecule such that when a mechanical force is applied, the change in the shape of the cage results in a change in resistance and, thus, can be detected via a change in current.
The sensor, which is 500,000 times smaller than a human hair, is able to deform at rates exceeding 800Hz, demonstrating its ability to be used repeatedly without failure. But where the researchers are particularly excited is that with the new sensor, it may be possible to explore more about junctions that form between molecules and attached metallic junctions.
As the new sensor is on the molecular scale, it falls under a new category of sensor called Nanoscale Electro-Mechanical Systems, or NEMS for short. While the device is far from being commercialised, it could very well provide the foundation for future NEMS devices.
Insights from the Researchers
The breakthrough in single-molecule sensing has not only captured the attention of the scientific community but has also ignited the enthusiasm of the researchers directly involved in the project. Dr. Nadim Darwish, a pivotal figure in this research, shed light on the broader implications of their findings. He articulated, "This new kind of piezoresistor could open up a whole new realm of opportunities for chemical and biosensors, human-machine interfaces, and health monitoring devices." His statement underscores the transformative potential of the single-molecule sensor in various domains, from healthcare to technology.
Echoing Dr. Darwish's sentiments, Professor Julian Gale, another key contributor to the research, emphasised the innovative approach they adopted. He remarked, "Detecting molecular shapes from their electrical conductance is a whole new concept of chemical sensing." This perspective highlights the departure from traditional sensing methods and underscores the novelty of their approach. The ability to detect molecular shapes through electrical conductance could pave the way for more precise and efficient sensing techniques in the future.
These firsthand insights from the researchers not only validate the significance of their work but also provide a glimpse into the future possibilities and applications of single-molecule sensors. As the field of nanotechnology continues to evolve, such pioneering research lays the foundation for advancements that could reshape industries and improve lives.
The Science Behind the Single-Molecule Sensor
In recent years, the realm of scientific research has been abuzz with discussions about the advancements in molecular sensing. Leading the charge in this domain is the groundbreaking research from Curtin University, which has provided a deeper understanding of the single-molecule sensor's functioning and potential applications.
According to a pivotal study published in Nature, the single-molecule sensor is not just a marvel of miniaturisation but also a testament to the precision of modern science. At its core, this sensor operates based on the principle of piezoresistance. But what does this mean? Piezoresistance refers to the change in the resistance of a material when mechanical stress or strain is applied to it.
The diaryl-bullvalene molecule, which is central to the sensor's operation, undergoes a significant structural change upon the application of mechanical force. This isn't just any change; it's a transformation that has measurable effects. Specifically, this alteration in the molecule's structure leads to a detectable change in its resistance. This phenomenon is what allows the sensor to achieve its primary function: precise sensing at the molecular level.
Such advancements hold immense promise for various fields, from medical diagnostics to environmental monitoring. As researchers continue to delve deeper into the intricacies of the single-molecule sensor, it's clear that we are on the cusp of a new era in molecular sensing, one that could revolutionise how we detect and understand the world at the tiniest scales.
Future Implications in Medical Diagnostics
The medical field is perpetually in pursuit of innovations that can enhance patient care, improve diagnostic accuracy, and ultimately save lives. In this context, the advent of the single-molecule sensor represents a significant stride forward, particularly in the domain of medical diagnostics.
The unique capability of the single-molecule sensor to detect subtle changes at the molecular level positions it as a game-changer in early disease detection. Imagine a world where diseases, even in their nascent stages, can be identified with unparalleled precision. This isn't just about identifying diseases earlier; it's about offering patients a fighting chance, often before symptoms even manifest.
Cancers, for instance, have always posed a formidable challenge to the medical community, primarily due to their often late detection. The single-molecule sensor, with its heightened sensitivity, could revolutionise cancer diagnostics. As underscored in a previous article, the benefits of early cancer detection are manifold. Not only does it significantly enhance the chances of successful treatment, but it also means less aggressive treatments, better patient prognosis, and a notable reduction in healthcare costs.
Beyond cancers, the sensor's potential applications span a wide array of medical conditions, from infectious diseases to genetic disorders. As research progresses and the technology matures, it's conceivable that such sensors could become an integral part of routine medical screenings, heralding a new era in proactive healthcare and personalised medicine.
How could such a device be revolutionary in the future?
While it is certainly impressive that the researchers were able to design such a sensor and be able to record the electrical activity from it, there are far wider implications to the development of this sensor.
One such case where this sensor could provide a massive leap in technological advancement is the detection of diseases and cancers. Simply put, diseases and cancers all comprise a unique, organic signature that is present on their outside (small chemical receptors), and it is possible to create enzymes that only react to these specific binding sites.
Thus, if the sensor developed by the researchers could be integrated with such enzymes, it becomes possible to detect the smallest presence of cancers and diseases. Not only would this help to catch diseases earlier, but also help simplify treatment as treatment options at earlier stages are often cheaper and easier to complete.
Another case of how this sensor could be major is in the development of new drugs. Again, if bound to enzymes, it becomes possible to detect how drugs react on a molecular level. This could help researchers identify causes of side effects while simultaneously being able to help manufacture patient-specific drugs that work on their specific biology.
Diving Deeper into Potential Applications
The single-molecule sensor, with its unprecedented sensitivity and precision, holds the potential to revolutionise a myriad of industries. Let's delve deeper into some of the most promising applications:
Environmental Monitoring
The ability to detect minute changes at the molecular level can be a game-changer for environmental monitoring. Whether it's detecting trace amounts of pollutants in water sources or monitoring air quality in real-time, the single-molecule sensor can provide data with unparalleled accuracy, enabling timely interventions and ensuring environmental safety.
Food Safety and Agriculture
In the realm of food safety, detecting contaminants, even in minuscule amounts, is crucial. The sensor could be used to identify the presence of harmful pathogens or chemicals in food products, ensuring consumer safety. Additionally, in agriculture, it could monitor soil health, detect pests, and even assess the quality of produce at the molecular level.
Security and Defense
The sensor's ability to detect specific molecular signatures can be harnessed for security applications. For instance, it could be used to detect trace amounts of explosive materials or chemical agents, providing an added layer of security in sensitive areas.
Research and Development
In scientific research, the sensor can be a valuable tool for studying molecular interactions, understanding material properties at the nanoscale, and even in drug discovery, where understanding molecular behaviours is paramount.
In essence, the potential applications of the single-molecule sensor are vast and varied. Its ability to provide insights at the molecular level, combined with its precision and sensitivity, positions it as a transformative tool that can reshape industries and enhance the quality of life.
Challenges Ahead and the Path Forward
In the realm of scientific innovation, every breakthrough is accompanied by its own set of challenges. The single-molecule sensor, despite its groundbreaking potential, is no exception.
From a technical standpoint, the intricacies of manufacturing and scaling such sensors are daunting. The miniaturised nature of these sensors makes them susceptible to interference from other electronic devices, potentially skewing results. Furthermore, their integration into existing systems presents another layer of complexity.
Economically, the initial stages of development and production might be accompanied by high costs, encompassing research, materials, manufacturing, and rigorous testing phases. Convincing stakeholders to invest in such a nascent technology could be a hurdle in itself.
Regulatory and ethical challenges loom large, especially when venturing into medical and environmental applications. Regulatory bodies will demand stringent proof of the sensor's safety, reliability, and accuracy. Ethical concerns, particularly around privacy and data security, could arise, especially if these sensors find their way into personal devices or public spaces.
Market acceptance is another mountain to climb. Industries and consumers, while intrigued, might approach such a novel technology with caution. Overcoming scepticism, educating potential users, and navigating resistance to change will be pivotal in ensuring the sensor's widespread adoption.
The interdisciplinary nature of this innovation necessitates seamless collaboration between experts from diverse fields. Physicists, chemists, biologists, engineers, and other professionals must come together, pooling their expertise to address the multifaceted challenges this sensor presents.
Lastly, questions about the long-term reliability and durability of these sensors remain. Their performance under varying conditions over extended periods, potential degradation, and maintenance requirements are areas that researchers must delve into.
In conclusion, while the path forward is riddled with challenges, the promise that single-molecule sensors hold is undeniable. With concerted efforts, collaboration, and a spirit of perseverance, the scientific community stands on the brink of ushering in a new era of sensing, with implications that could reshape industries and enhance the human experience.
References:
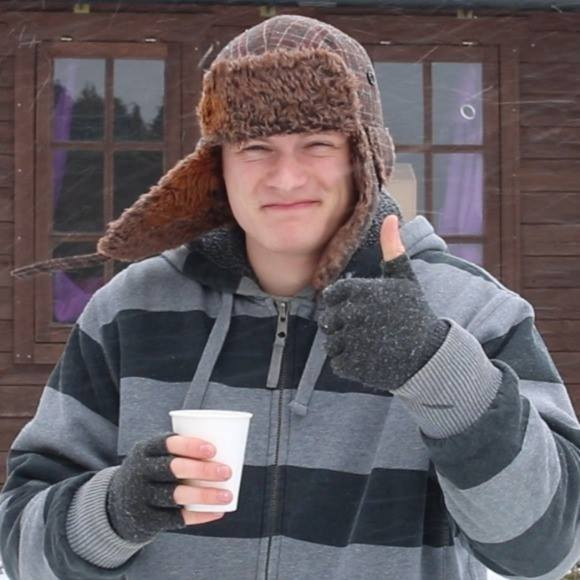