Triboelectric Fibres: Enhance Physiological Monitoring
16-05-2024 | By Liam Critchley
Textile electronics that use fibres have emerged as a system for monitoring human health and tracking fitness activities. In fibre electronics systems, a network of body area sensors is used to establish different sensing nodes that measure different physiological parameters. The ability to create low-power and self-powered sensors has emerged as a key factor in textile-based electronics.
Recent advancements in fibre electronics, particularly in the realm of triboelectric devices, have shown significant potential in transforming how we monitor and manage human health. Studies, such as the one published in Nature Communications, highlight the development of highly sensitive triboelectric micro-flexure sensors. These sensors, which draw inspiration from biological mechanisms, are capable of detecting minute physiological changes, thereby offering new possibilities for wearable health monitoring systems.
- Integration of Triboelectric Fibres: Recent advancements in triboelectric fibres offer significant potential for transforming health monitoring by detecting minute physiological changes, as demonstrated in the latest studies.
- Energy Harvesting: These fibres not only monitor physiological parameters but also harvest energy from the body's natural movements, leading to the development of self-sustaining wearable health monitoring systems.
- Applications in Healthcare: Triboelectric fibres can be used in smart textiles for continuous health monitoring, early detection of health issues, and personalised health management, including applications in sports, rehabilitation, and clinical settings.
- Biological Inspiration: Inspired by natural biological structures, triboelectric fibres mimic the sensitivity and adaptability of biological sensors, ensuring high performance even under varying environmental conditions.
Advancements in Triboelectric Technology for Health Monitoring
Triboelectric devices turn a strain caused by physical movement into an electrical charge. This has been seen most notably through the small-scale energy harvesters triboelectric nanogenerators (TENGs), and while they only produce a small current, it is often enough to power small-scale sensors—such as those that are used in smart textiles to monitor the body.
This innovative approach leverages the body's natural movements to generate energy, significantly enhancing the functionality of smart textiles. By integrating triboelectric fibres into fabrics, researchers have been able to create textiles that not only monitor various physiological parameters but also harvest energy efficiently. This dual functionality is a key step towards the development of self-sustaining wearable health monitoring systems.
In addition to energy harvesting, the sensitivity and adaptability of triboelectric fibres make them ideal for applications requiring precise physiological monitoring. For example, these fibres can detect subtle changes in body movements and physiological parameters with high accuracy, making them suitable for continuous health monitoring and early detection of potential health issues.
Applications of Triboelectric Fibres in Physiological Monitoring
Triboelectric devices take many forms, and one interesting area that is being looked at for physiological monitoring using e-textiles is the use of triboelectric fibres. These fibres could then harvest the natural body of the wearer and/or act as the sensing mechanism to provide an electrical response for different movements/motions, all while being the fabric of the clothes—saving on the need to integrate separate energy harvesters alongside the textile itself to power the integrated sensors.
One significant advantage of these fibres is their ability to function effectively in diverse environmental conditions. By mimicking the natural biological structures that excel in sensitivity and adaptability, triboelectric fibres can maintain high performance even under varying temperature and humidity levels, ensuring consistent monitoring accuracy.
The use of triboelectric fibres is seen a potential way to remove as many unnecessary components as possible from the textile, while still retaining its sensing functionality. There have been numerous studies dedicated to fibre-based sensors, but many have focused on high-strain applications, where there is a large mechanical deformation that causes a sensing response and/or an electrical current.
However, physiological movements from a human wearer are much smaller and are known as microflexures, and it is the measurement of these smaller motions that are of interest in biological monitoring applications. There have been many challenges to measuring such small deformations using triboelectric fibre-based sensors due to the intrinsic variability and subtle amplitude of physiological signals—all of which differ from person to person and from wearing environment to wearing environment. Researchers have looking to develop new triboelectric fibres that can meet the flexural demands of biological monitoring.
The Superiority of Biological Sensing Networks
It’s often said that nature is the best scientist/engineer and does things that we can’t, and it’s true. Millions of years of evolution have enabled nature to hone and perfect many different processes that humans can only dream of replicating artificially.
Biological sensory systems are superior to synthetic sensors and have a very high sensitivity towards small deformations—and can detect deformation in the range of 10 to 100 μm. They also have a very low threshold detection and can detect changes as small as 0.1 mm-1, and can dynamically adjust their structure and function to changes in the their local environment and the presence of external stimuli.
This ability to sense such small deformations means that pulsations, respiration, heartbeat, and muscle exertion can all be measured as the sensing threshold is sensitive enough to pick up the deformations. There are two factors that contribute to this sensing ability—the structure of the receptor and the signal feedback mechanism.
The receptor structure can vary in shape, but they share some common structures—such as layered stacking or coiling to amplify stimulus signals—and functional features which enhance the contact area for stimulation. These features enable receptors to respond to very slight deformations. One key example of this is Meissner corpuscles, which are mechanoreceptors that have cutaneous nerve endings which transmit the sensations associated with touch and vibration.
The ion conduction mechanism of biological sensor systems is also susceptible to weak field changes for detecting small potential differences. This is a feature of biological sensing systems that ensure a high-quality signal transmission. All the structural and functional features enable biological sensors to be highly sensitive, and researchers have been looking to emulate these characteristics to create artificial triboelectric fibre sensors that are suitable for physiological monitoring.
For instance, a recent study published in Nature Communications described the development of triboelectric fibres that incorporate an ordered distribution of nanofibre buckling at the ionic conductive interface. This design significantly enhances the fibres' ability to detect and respond to minute physiological signals, such as those produced by muscle contractions and subtle body movements.
Moreover, recent research has demonstrated that incorporating nanofibre buckling structures into triboelectric fibres can significantly enhance their sensitivity and robustness. This approach not only improves the fibres' ability to detect microflexures but also ensures their durability over extended periods of use, making them more reliable for long-term health monitoring applications.
Taking Biological Inspiration to Build Artificial Triboelectric Fibres
Researchers have now taken inspiration from the Meissner corpuscle and have created a microflexure-sensitive fibre. The sensing fibre is a sheath-core fibre where an ordered distribution of buckled nanofibre structures is implemented at an ionic conductive interface.
In a Meissner corpuscle, the cleft space and lamellar cells are responsible for the deformation detection through mechanical compliance. In a similar vein, the triboelectric fibres show similar principles. High surface area tribo-interfaces were constructed in the fibre by twisting the nanofibers with different modulus ratios on to the surface shape memory hollow fibres. Annealing processes were also used to maximise the tribogap within the structure.
Both structural aspects of the fibre mimicked the Meissner corpuscle’s function and were responsible for a range of advantageous properties in the fibre. This includes an enhanced sensitivity, where a detectable signal can be recorded at ultra-low curvatures of 0.1 mm-1 with a flexure factor of about 21% and a bending range of 10°. The other functional property that this structure brings is a mechanical robustness under microflexure, which enables the fibres to undergo more than 10,000 bending cycles.
To illustrate the practical applications of triboelectric fibres in health monitoring, recent research published in Nature Communications demonstrates how these fibres can be used to monitor subtle physiological activities. The study highlights the potential of triboelectric fibres to detect and measure minute deformations in real-time, providing valuable data for health diagnostics and monitoring. Below is an example of how these fibres are implemented for various physiological measurements:
a The schematic diagram shows the placement of the fiber sensor on the arm arteries and muscle groups of the upper extremity, detailing the detection principle for monitoring muscle and artery contractions and relaxations. b Depictions of microscopic physiological activities within the human body and a statistical analysis of the deformation range of various tissues. c The triboelectric mechanism used by NB-fiber for pulse detection is explained. d Interpretation and display of pulse waveforms extracted during exercise and rest. e Analysis of various parameters such as Augmentation Index (AIx), ejection time, and pulse waveform frequency in different states, including resting, walking, and running. The top panel illustrates the variation in pulse frequency obtained through Fourier transform analysis. f A Poincaré plot shows the pulse detection over a 30-second duration in a 22-year-old male. g Measurement of the electrical signal amplitudes corresponding to muscle strength under different motions. h A schematic diagram of the sensor for pulse and respiration monitoring is provided. i Extraction and interpretation of respiratory waveforms in different states.
Furthermore, the robustness of these fibres under repeated mechanical stress makes them ideal for use in wearable devices that require long-term durability. This feature is particularly beneficial for applications in sports and rehabilitation, where continuous monitoring over extended periods is essential.
Long-Term Durability and Applications in Healthcare
The advanced properties of these triboelectric fibres open up a wide range of applications in healthcare. For instance, they can be integrated into smart clothing that not only monitors physiological parameters but also provides real-time feedback to the wearer, enhancing the effectiveness of health and fitness routines. This integration can lead to more personalised and data-driven approaches to health management.
The combination of flexibility in the fibres means that they can be used in muscle force detection cuffs that provide monitoring of various muscle groups, postures, and force feedback. The fibres can also be used to monitor many subtle physiological diagnoses, including upper limb muscle strength training and improving the power control’s precision of muscles during rehabilitation. The fibres can also be used to track and analyse movement patterns in physical therapy to provide more personalised and effective treatments. There’s even the possibility of adapting the fibres for advanced prosthetics and exoskeletons for people with mobility impairments.
Additionally, the use of these fibres in clinical settings could transform patient care by providing continuous, non-invasive monitoring of vital signs. This capability is particularly valuable for patients with chronic conditions, enabling healthcare providers to track their health status in real-time and respond promptly to any adverse changes.
Overall, the development of triboelectric fibres that can measure minute deformations and physiological changes opens the fibres for use in a wide range of healthcare and wellness applications.
Reference:
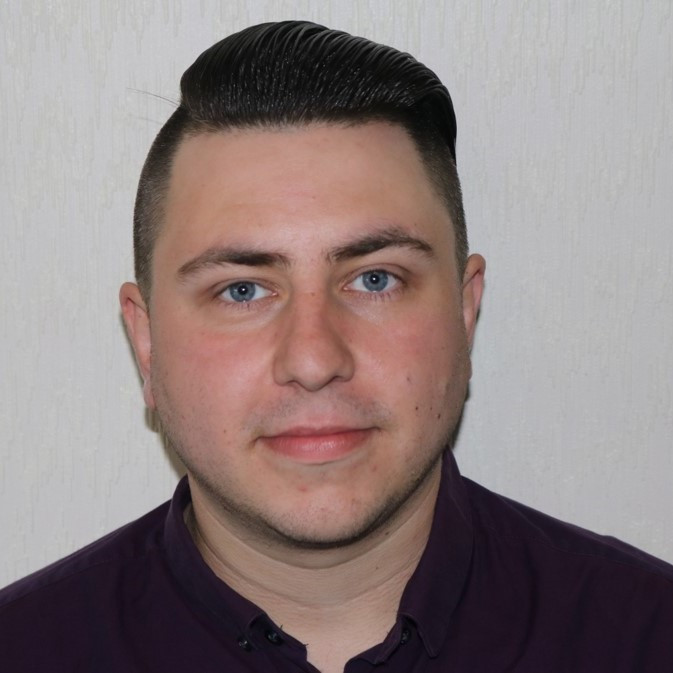