Taming Wind and Sun with Silicon Carbide & GaN
24-06-2024 | By Gary Elinoff
Key Takeaways about Silicon Carbide and Gallium Nitride Tame the Wind and the Sun:
- The wind's speed and the sun's intensity at ground levels are, of course, highly variable. As a result, the electrical outputs of wind turbines and solar farms are not steady or even predictable.
- DC that varies in amplitude or AC that varies in either amplitude or frequency cannot be used by the power grid.
- Silicon Carbide (SiC) and Gallium Nitride (GaN) Semiconductors are the key elements in harnessing that raw, untamed power and rendering it into a useful form.
Introduction
Solar cells convert the sun's energy into electricity, and wind turbines generate electricity from the wind. But the sun doesn't always shine, and when it does, its intensity at any given patch of earth will vary wildly. The same can be said for the capricious nature of the wind. Both will vary with the time of year, the time of day and, of course, the specific local weather conditions. Consequently, the nature of the electricity provided by solar farms and wind turbines is not to any degree predictable.
But that won't work for power line transmission.
Power lines usually carry AC voltage or, occasionally, DC. However, in either case, they only carry specific voltage levels, and in the most typical case of an AC power line, it must also have a very specific frequency – 60 cycles per second in the USA.
And there's the core of our problem. So, before that wonderful, non-polluting renewable energy is of any use, it must be corralled, tamed and made to conform with the power line's requirements.
OK – so How to Tame Wild, Unpredictable Renewable Energy?
There are some central similarities between converting DC to AC, AC to DC and changing voltage levels. The source voltage must be presented to a semiconductor switch, which must open or close many thousands of times per second, yielding voltage "pulses", which can be collectively described as pulsating DC. From there, a variety of modalities can be deployed to get the specific power pattern that's needed by a specific power line. For now, let's first look at the types of semiconductors employed to do the job. These are SiC and GaN, the Wide Bandgap semiconductors.
What are Wide Bandgap Semiconductors?
Wide bandgap semiconductors can switch "ON" and "OFF" at extremely fast rates, and of great importance, when they turn "ON", they offer very little electrical resistance. They can also handle more power. Additionally, not only can they operate safely and efficiently at higher temperatures, but they also transfer heat more efficiently.
Let's compare SiC, GaN and classical Silicon devices. Keep in mind that vast amounts of money world wide is being spent on power semiconductor research, and the diagram below must be considered as only a snapshot of a moment in time.
Silicon Semiconductors vs GaN Vs SiC. Image source: Texas Instruments
What's apparent is that while SiC can handle more power than SiC, GaN can switch faster. GaN is also less expensive. GaN's operational power levels, as described in a previous Electropages article, are often enough to convert an EV's main battery's DC into AC for the vehicle's main motor. However, given the multi-megawatt scale of solar farms or wind turbine systems, SiC is the preferred wide bandgap semiconductor for the task of dealing with utility-scale renewable energy.
How Does an Inverter Work?
Let's look at the base case, converting the DC derived from a solar panel into AC. The device that accomplishes this most fundamental step is known as an inverter. Residential scale solar inverters typically employ GaN, because the power (and added expense) of SiC is not needed.
The basic idea behind an inverter. Modified image source: The engineering mindset
In this very simplified illustration, imagine S1 and S4 turning "ON" while S2 and S3 stay "OFF". The voltage from the battery's positive terminal on the top flows through S1, into the light bulb's left side, and out the bulb's right side back to the battery's negative terminal on the bottom.
Next, imagine that S1 and S4 turn "OFF" and S2 and S3 turn "ON".
This time, the situation is reversed. The voltage from the battery's negative terminal on its bottom flows through S3, into the light bulb's left side, and out the bulb's right side back to the battery's positive terminal on the top.
Note that at first, the lightbulb's left side receives a positive voltage. Then, when the switches change, it gets negative voltage. Thus, with the bulb's left side first hit with positive voltage and then hit with negative voltage, a simple "AC" pattern is generated. Of course in real life, the switches are replaced by semiconductor switches, whose turn on and turn off sequences are determined by microcontrollers.
When SiC or GaN semiconductors are employed, little power is wasted across the "switch" because WBG semiconductors offer little resistance in the "ON" state. What little heat is generated is well tolerated and easily dissipated. And when higher frequency AC is required, WBG semiconductors can do the job better than last-generation silicon can.
A Typical Energy Flow From Sun to Grid
But it's not that simple, and that's because the constantly varying power of the sun's rays as they impact the solar panels produce constantly varying electrical output. The illustration at the head of this article illustrates the situation in a very basic manner. The sun's ever-varying intensity produces fluctuating DC, and the DC/DC converter stabilizes it.
Most basic DC/DC converter.
The switch in the above design is, of course, a semiconductor switch. The final step in this basic illustration is the DC-AC inverter, which is a sophisticated version of the simplistic inverter presented in the last section.
Challenges and Opportunities
While GaN is fast improving in power level capacity, SiC is the odds-on champion, and they are the choice for utility-scale renewable energy systems. Thanks to these devices, energy efficiencies from the sun and wind to the grid are reaching unprecedented levels.
A pressing problem is the cost of SiC. But these semiconductors are a sought-after element for a wide variety of military and civilian applications, and because of this need, manufacturers worldwide are pressing ahead with SiC research and, most importantly, with improving yields in the foundries.
Wrapping Up
Because of the ever-shifting intensity of sun and wind and the exacting requirement of the power grid, multiple voltage conversions from AC to DC, DC to AC, as well as changing voltage levels as well are required to properly feed the power grid.
SiC and GaN offer tremendous advantages over last-generation silicon-based semiconductors. Employed as switches as they are in voltage conversion devices, their high switching speeds present the opportunity of creating very fast DC pulses, a great advantage, for example, in building smaller, lighter, more efficient converting devices. Their lower resistance when they switch "ON" means less power is wasted within the semiconductor. And WGBs can operate at higher temperatures than can classical silicon devices, so whatever waste heat is generated can be better tolerated.
These conversions can be done with classical silicon devices, but they can be done with far greater efficiency with SiC and GaN, the WGB semiconductors.
References:
- Silicon Carbide enables smaller, more efficient large-scale solar: https://www.wolfspeed.com/applications/power/renewable-energy/solar-energy-systems/industrial-commercial-solar-systems/
- 2. How silicon carbide helps maximize efficiency in renewable energy systems: https://www.ti.com/document-viewer/lit/html/SSZTCY2
Glossary of Key Terms:
- Wide Bandgap Semiconductors (WBG). A type of semiconductor that switches faster offers less electrical resistance and can operate safely at greater temperatures than classical silicon-based devices can.
- Inverter. A device whose function it is to convert DC voltage into AC voltage
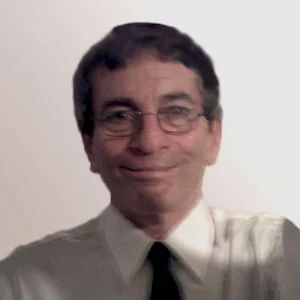