Liquid Crystal Adaptive Electronics: Advancing Interactive Systems
16-07-2024 | By Liam Critchley
Scientists and engineers are always taking inspiration from nature to build new advanced electronics systems. Many things have been achieved with artificial electronics thanks to the natural world around us, but one that has been a long-standing goal to mimic is adaptive behaviour in artificial systems that are analogous to natural systems.
Recent advancements in adaptive electronic systems have showcased the potential of integrating natural mimicry into synthetic materials. Studies, such as those exploring the behaviour of liquid crystal networks, have demonstrated the feasibility of creating systems that can autonomously adapt to environmental changes, akin to natural organisms.
Key Things to Know:
- Recent advancements in adaptive electronic systems have demonstrated the potential of integrating natural mimicry into synthetic materials, achieving responsive behaviour akin to natural organisms.
- Research highlights the development of liquid crystal network-based adaptive electronics, which integrate sensing, signal processing, and actuating functions into a single cohesive system.
- These systems can autonomously adapt to environmental changes, offering new possibilities for advanced electronic applications and improving functionality and efficiency.
- The innovative use of liquid crystal polymers in adaptive electronics represents a significant leap forward in creating more intelligent and autonomous devices, paving the way for future applications in self-learning systems and soft robotics.
Advancements in Adaptive Electronic Systems
Adaptive behaviour is a complex but vital trait in living systems (both animals and plants) that enables them to independently process and respond to environmental stimuli using a closed-loop feedback mechanism. These natural systems rely on efficient sensing, signal processing, and actuating functions to facilitate this adaptive behaviour.
For example, research published in Nature Communications highlights the development of adaptive units based on liquid crystal oligomers that seamlessly integrate sensing, processing, and actuating functions. This integration mimics the natural feedback mechanisms seen in plants and animals, offering new possibilities for advanced electronic applications.
A lot of efforts to date to realise adaptive capabilities in synthetic electronics via biomimicry try to follow the natural blueprint by using sensors, an external controller and actuators. However, many engineers have faced a lot of challenges when trying to combine all three of these elements together into a single functional system that can independently cope with dynamic environments.
Researchers have now taken a new approach to solving the issues around the components not working together by building an electronic unit based on a liquid crystal polymer that can incorporate sensing, signal processing, and actuating functionalities without issue.
One innovative solution involves the use of liquid crystal network-based adaptive electronics, which provide a unified platform for these functions. This approach, detailed in recent studies, leverages the unique properties of liquid crystals to achieve responsive behaviour that closely emulates biological systems.
Attempting to Mimic the Functions of Plants
One example in nature of a living organism that exhibits a high level of adaptability is the Mimosa Pudica Linn plant. The leaves of this plant fold when they are exposed to specific environmental stimuli, such as touch, changes in temperature, and light. The dynamic response from the plant to fold the leaves protects the plant from herbivores. The plant senses environmental signals, processes the data from these sensing mechanisms, and generates a physical response—all of which are used to create a functional feedback loop.
Researchers have utilised similar concepts in adaptive electronics, creating systems that can respond dynamically to environmental stimuli. This approach enhances the functionality and efficiency of artificial systems, making them more robust and versatile in real-world applications.
While there have been efforts to mimic these types of feedback loop systems, a lot of approaches have centred around developing centralised control systems that use extra controllers to interface with the different sensing and actuation components. However, these components are typically made of various materials using different fabrication methods, meaning that they are all manufactured separately.
The challenge of material property mismatches can be mitigated by adopting a more integrated fabrication approach. For instance, the use of liquid crystal networks allows for the cohesive integration of multiple functionalities within a single material, streamlining the manufacturing process and improving overall system performance.
Because they are created separately, it leads to material property mismatch that causes variations in signal strength and prevents the different functional elements from acting like a single, unified, and cohesive unit. Centralised processing methods also limit the capability of the system because they are limited when it comes to processing the large amounts of data that are generated by the sensors in artificial systems. Developing a new system that can bring all these elements together has been a pressing matter for developing new adaptive electronics.
Building Artificial Adaptive Electronics based on the Mimosa Plant
Researchers have now developed a new adaptive electronic system that achieves the seamless integration of the three main components—sensing, signal processing, and actuating—and can transfer information using closed-loop feedback. The system combines multiple adaptive units to perform ‘sense-decide-act’ operations in response to stimuli.
Design Strategy and Fabrication
The design strategy of these adaptive electronic units involves a combination of advanced materials and innovative fabrication techniques. As illustrated in Figure 1, the adaptive unit mimics the response of the Mimosa pudica Linn’s leaves to environmental stimuli. This approach ensures that the electronic unit can autonomously adapt to changes, much like natural organisms.
Figure 1: a Left, schematic showing the response of Mimosa pudica Linn’s leaves to human touch. Right, a printed adaptive electronic unit mimicking a Mimosa leaflet on a human finger. The background shows birefringence colour of the printed LCON film under a polarized optical microscope. Scale bar: 300 µm. b 3D illustration of the direct ink writing method for fabricating an LCON adaptive unit. Enlarged images explain the principle of (i) a self-propelled gate (SPG) closing the gap in the conductor during LCON heating, and (ii) a self-terminated gate (STG) interrupting the conductor by heating. Red arrows indicate the direction of internal stress. Tth denotes the threshold temperature. c 2D-WAXD measurement of an LCON at room temperature, suggesting a high scalar order parameter (S) in the oligomeric network. d Measurement of thermal expansion coefficient (α) as a function of temperature. The anisotropic thermal expansion α of LCON is measured perpendicular to the director (⊥) as shown by the blue line, and parallel to the director (//) as indicated by the red line. The green line represents the α of the electrode. e Scanning electron microscopy (SEM) measurements of a micro-gap of about 6 µm created in the SPG at room temperature. Scale bars indicate 100 µm and 30 µm in the left and right images, respectively. f Resistance change as a function of relative temperature Tac/Tth (°C/°C). Tac represents the actual temperature in the LCON. The green line denotes SPG, while the red line indicates STG. Both finite element modeling (FEM) results and experimental results are represented by solid dots and hollow dots, respectively.
Overview of the Adaptive Electronic System
This innovative system, detailed in Nature Communications, employs advanced materials and design strategies to create highly efficient and responsive adaptive units. By integrating these components into a single, cohesive system, researchers have paved the way for more advanced and reliable adaptive electronics.
This new system is based on a liquid crystal polymer—a state of matter that is between a liquid and a solid where it can flow like a liquid, but the individual molecular constituents can be orientated like a solid material. This approach was inspired by the macroscopic response of the Mimosa plant.
The system is composed of different adaptive electronic modules that integrate sensing, signal processing, and actuating functionalities, all in a single material. The system converts the stimuli—including heat, light, and touch—and changes it into an electrical signal that enables adaptivity to dynamically changing environments. To achieve this, the polymer molecules form a film and undergo anisotropic deformation in the presence of a stimuli used.
The ability to convert various stimuli into electrical signals is a significant advancement in adaptive electronics. This capability allows the system to maintain high performance and reliability across a range of environmental conditions, making it suitable for diverse applications.
Key Components and Functionality
The system contains four fundamental components that enable the system to function adaptively. The core element is the liquid crystal network that provides sensing and actuating functions and is supported by two functional electrodes. The first electrode is a sensing electrode that is located within the liquid crystal film and allows the transmission of electrical signals across the device when environmental changes induce deformation in the liquid crystal—and like many sensing systems, the deformation causes an electrical change in the liquid crystal that is transmitted and detected, enabling adaptive actions to be implemented.
The actuation is facilitated by both the liquid crystal and the second electrode—which is an actuating electrode that converts electric signals into thermal signals via Joule heating to facilitate actuation and movement in the device. The actuation electrode is located at the edge of the liquid crystal and connected in series with the sensing electrode and a power source—with the power source only being an energy input and not for electrical control.
The adaptive system was applied to facilitate dynamic interactions between units or between units and their local environments. By changing the orientation of the molecules in the liquid crystal between parallel and perpendicular to the sensing electrode, the device could operate under two binary logic operations—which are the self-propelled gate (SPG) and self-terminated gate (STG).
These dynamic interactions are crucial for the development of next-generation electronic systems. The integration of adaptive units capable of 'sense-decide-act' operations represents a significant leap forward in creating more intelligent and autonomous devices.
In the device the SPG initiates signal transmission and generates a positive feedback loop, and the STG stops the signal transmission to create a negative feedback loop. The signal transmission speed is on the order of seconds—which is comparable to the Mimosa plant—and the closed loop means that large swathes of data produced by the sensors are not an issue like in many other systems. The researchers also created an artificial Mimosa plant using their adaptive electronics that exhibited the same folding response as the real Mimosa when touched.
Embedding the sensors into a responsive material that also acts as part of the sensing and actuating functions has helped to build a system where all the key adaptive components can be integrated together and work.
Advantages and Future Applications
This integration reduces the complexity and potential points of failure within the system, resulting in a more robust and efficient design. The use of liquid crystal networks, in particular, has shown promise in achieving these goals, as highlighted in recent scientific publications.
This advancement in flexible electronics and the ability to handle higher computational functions could open the door to this system being used in multiple adaptive applications, including self-learning intelligent systems, swarm intelligence and soft robotics.
Reference:
Lyu, P., Broer, D.J. & Liu, D. Advancing interactive systems with liquid crystal network-based adaptive electronics. Nat Commun 15, 4191 (2024). https://doi.org/10.1038/s41467-024-48353-7
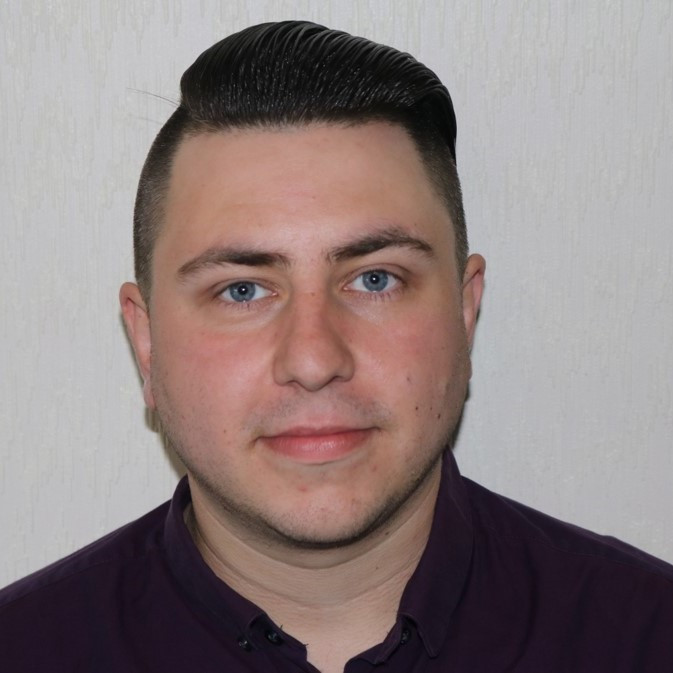