NASA Demonstrates Ultra-Cool Quantum Sensor in Space Milestone
06-09-2024 | By Robin Mitchell
NASA’s Cold Atom Lab, pictured aboard the International Space Station, recently demonstrated an atom interferometer, a tool capable of precisely measuring gravity and other forces with numerous space applications. Credit: NASA/JPL-Caltech
NASA has achieved a groundbreaking milestone by successfully demonstrating an 'ultra-cool' quantum sensor in space for the first time, as reported by Phys.org. This cutting-edge quantum sensor, known for its extreme sensitivity and ability to detect the faintest electromagnetic signals, opens up new possibilities for space exploration and scientific research. With this remarkable achievement, NASA is paving the way for unprecedented advancements in space technology and exploration. What challenges do ultra-cool sensors face when operating in space, how will the deployment of the 'ultra-cool' quantum sensor revolutionise space research and exploration missions, and how does the sensor's performance in space compare to its capabilities in controlled laboratory settings?
Key Things to Know:
- NASA has successfully demonstrated the first 'ultra-cool' quantum sensor in space, a breakthrough in space exploration technology.
- The quantum sensor's extreme sensitivity opens new possibilities for detecting electromagnetic signals in space, enhancing scientific research and exploration missions.
- Challenges in operating ultra-cool sensors in space include extreme temperature fluctuations, cosmic radiation, and maintaining quantum coherence.
- The Cold Atom Lab's atom interferometry could help uncover cosmological mysteries like dark matter and dark energy, and provide new insights into planetary compositions.
Space Detectors Face Major Hurdles
As humans, we have always been fascinated with the night sky, and the idea of gazing up at the stars sparked all kinds of innovation. The development of telescopes allowed us to see the intricacies of the universe, while the invention of satellites enabled us to have technology up in the heavens. But as technology advanced, so did our desire to observe the universe with the creation of ultra-cool sensors that can see the faint light from distant galaxies and black holes.
Despite the many advances made, these ultra-cool sensors still face numerous challenges that hinder their performance. Operating in space, these sensors need to be able to see through the infrared light emitted from Earth and the sun and yet be able to distinguish the smallest changes in temperature. The extreme distances between objects also mean that only so much information can be detected, while the interference from planets and other celestial bodies further reduces the capabilities of such sensors. Thus, humans have turned to developing arrays of detectors and advanced processing systems to try and make the most of these sensors, but the challenges remain.
The space environment is unforgiving, and ultra-cool sensors face numerous difficulties when deployed in space. The vacuum of space results in a lack of thermal mass, and this makes cooling down rapidly challenging. The extreme temperature fluctuations also include the cold side of space, which can see sensors rapidly losing their quantum coherence, and this, in turn, affects their performance. The high-energy particles also include cosmic rays, and these can damage sensitive electronic components, including sensors. From there, the extreme conditions of space can also include strong vibrations and micro-impacts from debris, and this also has the potential to damage sensors.
Challenges Facing Ultra-Cool Sensors in the Harsh Space Environment
From the point of view of electronics, ultra-cool sensors also face challenges with power consumption and miniaturisation. The use of smaller devices helps to reduce weight, which is advantageous for space travel, but this also means that sensors have reduced processing capabilities and smaller memory spaces. This, in turn, affects their ability to pre-process data before sending it back to Earth, and this can put additional pressure on ground-based systems for data analysis.
Maintaining the state of ultra-cool sensors in space also presents challenges with data transmission. The vast distance between Earth and space makes communication challenging, and the weak signal strength means that data transmission errors are common. As such, ultra-cool sensors need to integrate strong communication systems to ensure that data is transmitted reliably.
Finally, the extreme environment of space also takes its toll on equipment, and ultra-cool sensors are no exception. The constant exposure to extreme temperatures, radiation, and other harsh conditions can damage the sensors over time, and this means that they need to be replaced. However, replacing sensors in space is a difficult task, as space is far from reach. Thus, cool sensors in space need to be designed with maintenance in mind, or else they will quickly cease to operate effectively.
Space Exploration Quantum Sensor Milestone Achieved by NASA
Recently, NASA has achieved a major milestone by successfully demonstrating an ultra-cool quantum sensor in space for the first time. This cutting-edge quantum sensor, which is extremely sensitive and can detect the faintest electromagnetic signals, is now open to new possibilities in space exploration and scientific research. With this achievement, NASA is paving the way for unprecedented advancements in space technology and exploration.
Breakthrough in Quantum Sensing Technology for Space Exploration
The research team utilised an atom interferometer, a quantum device capable of precisely measuring gravity, magnetic fields, and other forces. On Earth, atom interferometry is used to explore fundamental gravitational forces and develop technologies for aircraft and ship navigation. However, applying this technique in space, where microgravity allows for extended measurement times and heightened sensitivity, has long been a challenge due to the fragility of the equipment.
The Cold Atom Lab, operated remotely from Earth since its launch in 2018, has overcome these challenges, demonstrating the feasibility of long-term quantum experiments in space. "Reaching this milestone was incredibly challenging, and our success was not always a given," said Jason Williams, Cold Atom Lab project scientist at NASA's Jet Propulsion Laboratory.
The ability to measure gravity with high precision in space has vast implications. Space-based sensors like those on the Cold Atom Lab could offer new insights into the composition of planets and moons by detecting subtle gravitational variations. These measurements, akin to those performed by the U.S.-German GRACE-FO mission on Earth, could reveal intricate details of surface mass changes and enhance our understanding of planetary bodies.
Furthermore, the utilisation of atom interferometry in the Cold Atom Lab could enhance our understanding of Earth's gravity field by detecting anomalies that may point to sub-surface geological formations. The sensitivity of these sensors in microgravity environments is particularly advantageous for planetary exploration, as they can operate without interference from atmospheric or surface vibrations.
Moreover, the atom interferometer's precision could shed light on cosmological mysteries such as dark matter and dark energy. These phenomena, which drive the universe's structure and expansion, remain largely elusive. "Atom interferometry could also be used to test Einstein's theory of general relativity in new ways," said Cass Sackett, a professor at the University of Virginia and a Cold Atom Lab principal investigator. "This technology may help us fill in those gaps and give us a more complete picture of the reality we inhabit."
Unlocking the Mysteries of the Universe with Atom Interferometry
The precise nature of atom interferometry has already shown promise in exploring fundamental questions in quantum mechanics, such as the potential to measure gravitational waves directly in space. This capability, when combined with Earth-based interferometry systems, could offer unparalleled insights into the behaviour of gravitational forces on both macro and quantum scales.
The Cold Atom Lab, roughly the size of a minifridge, operates at near absolute zero, allowing scientists to study Bose-Einstein condensates—a state of matter where atoms share a single quantum identity. This environment makes it possible to observe quantum properties on a macroscopic scale, advancing our understanding of how matter behaves at the quantum level. In microgravity, these condensates reach colder temperatures and persist longer than on Earth, providing more opportunities for in-depth study. The atom interferometer is one of several tools in the Cold Atom Lab that harness the quantum nature of atoms for precision measurements.
Nick Bigelow, a professor at the University of Rochester and a principal investigator for the Cold Atom Lab, emphasised the broader implications of this work. "I expect that space-based atom interferometry will lead to exciting new discoveries and fantastic quantum technologies impacting everyday life, and will transport us into a quantum future."
This quantum future extends beyond space exploration, as the atom interferometry methods refined in space could influence advancements in telecommunications, medical imaging, and even geophysics. By improving the precision of quantum sensors, industries on Earth could develop more accurate diagnostic tools, enhancing fields like healthcare and environmental monitoring.
Space Age Quantum Revolution
Quantum sensors have long been hailed as exceptional in controlled laboratory environments, but their true test is in the challenging environment of space. Once deployed outside of Earth labs, their potential to revolutionise space exploration becomes apparent.
The extreme conditions of space, including microgravity and cosmic radiation, do not hinder but instead enhance the functionality of quantum sensors. The sensor's ability to operate in the harsh environment of space demonstrates their resilience and adaptability, proving their reliability for future space missions.
The use of quantum sensors in space opens up many possibilities for engineers and scientists. By leveraging the sensor's enhanced performance, engineers can develop more advanced navigation systems for spacecraft, enabling precise manoeuvring and accurate positioning in deep space. The improved measurement precision also facilitates environmental monitoring in space, allowing for a better understanding of celestial bodies and their interactions.
Quantum sensors also pave the way for new discoveries in astrophysics and cosmology. Their unparalleled sensitivity enables scientists to detect faint signals from distant stars and galaxies, providing insights into the mysteries of the universe. With enhanced measurement capabilities, researchers can delve deeper into the complexities of space and unravel the secrets of the cosmos.
The technological impact of quantum sensors in space extends beyond space exploration, driving the development of new technologies and engineering solutions for various industries on Earth. The high precision and sensitivity of quantum sensors inspire innovation in diverse fields, leading to the creation of cutting-edge devices and systems that revolutionise modern technology.
Looking ahead, NASA plans to integrate quantum sensors into future missions to enhance scientific observations and improve spacecraft navigation. By harnessing the power of quantum sensors, NASA aims to unlock new frontiers in space exploration and pave the way for groundbreaking discoveries.
In conclusion, the performance of quantum sensors in space far exceeds their capabilities in labs, showcasing their potential to revolutionise space exploration. Engineers and scientists will benefit greatly from the enhanced performance of quantum sensors, enabling unprecedented advancements in navigation, environmental monitoring, research, and technology. With NASA leading the way in integrating quantum sensing technologies into future missions, the future of space exploration looks brighter than ever before.
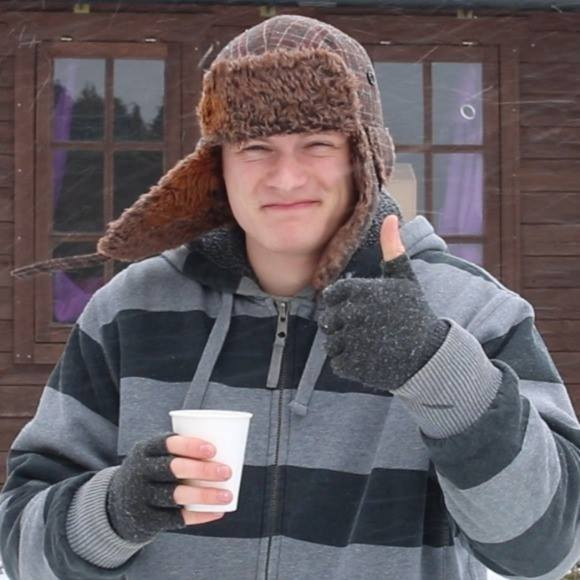