Graphene Electrical Nanopatches Enhance Brain Injury Recovery
03-10-2024 | By Liam Critchley
Key Things to Know:
- Traumatic brain injury (TBI) is a leading cause of death and disability worldwide, with no effective treatments for repairing damaged brain tissue due to limited neuron regeneration capacity.
- Neural stem cell (NSC) therapies show promise in repairing damaged brain tissue, but challenges remain in directing transplanted stem cells to differentiate into functional neurons.
- Graphene nanopatches offer a breakthrough by providing continuous electrical stimulation to migrating stem cells, enhancing their ability to differentiate into neurons and potentially treating TBI.
- Recent research demonstrates that graphene-wrapped stem cells significantly improved brain recovery in animal models, opening new possibilities for treating neurodegenerative diseases and nerve injuries.
Introduction
Traumatic brain injury is a leading cause of disability and death around the world. TBI is caused by mechanical damage to the brain, which triggers a range of cortical network abnormalities—such as neuron death and synaptic circuit damage. There are currently no effective treatments available for repairing brain tissue because this tissue has a very limited capacity to produce new neurons after an injury has occurred.
Neural stem cell (NSC) therapies have shown some potential to rebuild neuronal circuits in the brain and restore brain tissue by replacing damaged neurons with new neurons formed from the stem cells. Implantation of NSCs has shown some limited success, but the ability of the transplanted cells to differentiate and be directed into functional neurons within the injury sites has been a problem.
It’s been established that electrical stimulation holds a lot of promise for enhancing neuronal differentiation of NSCs to treat TBI. However, current approaches have faced their own challenges; namely, when the stem cells leave the electrically stimulating material and migrate, the electrical stimulation is removed, and issues around not being able to direct neurons into different functions resurface. Researchers have now turned to wrapping up stem cells in graphene nanopatches to try and solve this challenge and create a therapy where the stem cells can differentiate properly under continued electrical stimulation.
Pulsed Electrical Stimulation Seen as Key TBI Therapy
Pulsed electrical stimulation is seen as a key strategy for treating brain injuries, and that the electrical stimulation can promote neural differentiation of stem cells by activating cytomembrane receptors, ion transport channels, or intracellular communication. However, electrical stimulation typically requires electrodes to be implanted with wires connecting the electrodes to an external power source. Approaches like this carry a risk of injury, as well as increased inflammation and infection from the surgical procedure and the presence of the implanted electrodes inside the body. It’s also difficult to introduce both the electrodes and stem cells to the target area, making it difficult to precisely apply electrical stimulation to individual cells.
Using wireless electrical stimulation has recently gathered a lot of interest as a way of overcoming the issues with bulky electrodes. Electromagnetic induction and the piezoelectric effect are some examples of wireless electrical stimulation to date. While they have so far been capable of providing a wireless electrical stimulation, none of the approaches to date have managed to stimulate individual stem cells, and some approaches can harm non-target cells.
Turning to Graphene to Generate Electrical Signals
Researchers have turned to a material with one of the best electrical properties in the world—graphene. There’s often some scepticism around using graphene in the healthcare sector, but graphene has already been used in a range of medical devices—in both external monitoring devices and implantable medical devices (IMDs).
In this latest approach, researchers wrapped NSCs in graphene nanosheets to form an electrical graphene nanopatch. Under electromagnetic induction, the conductive graphene nanosheets deliver an electrical stimulation. Because the NSCs are wrapped in between the sheets, the nanopatch can deliver in-situ electrical signals to individually stimulate the neuronal differentiation of NSCs and use it as an effective TBI therapy.
This approach means that when the NSCs are migrating, they are still involved with the stimulating material, so they can be stimulated on-demand. The ability to undergo electromagnetic induction enables the nanopatch to generate periodical pulse electrical signals and apply them to target cells in the presence of a periodic magnetic field.
The amplitude and frequency of the electrical stimulation can be controlled by changing the rotation speed of the magnetic field. The nanopatches were also modified with a layer of laminin that tightly wrapped the cell membranes into the patch, so when the magnetic field was changed, it electrically stimulated the neuronal differentiation of stem cells. The wrapping of the NSCs was found to be an important factor because 41% of nanopatch-wrapped stem cells differentiated into functional neurons within 5 days compared to only 16.3% of unwrapped ones.
Previous studies have discovered that changing the magnetic field itself could electrically stimulate the neuronal differentiation of stem cells. However, this requires long stimulation times of over 4 hours a day or large magnetic field strengths of at least 0.83 T. In these scenarios, the number of differentiated stem cells only increased 1.6-fold. In comparison, the nanopatches only require 15 minutes a day under a low magnetic field strength of 0.16 T to increase the differentiation by 2.6-fold. Additionally, high magnetic field strengths will apply electrical stimulation to all tissues and cells exposed to the magnetic field, potentially causing side effects—something which was negated by the nanopatches and low magnetic field strength.
The researchers tried the nanopatch on a mice model and anchored them to a lesion site. The nanopatch was exposed to a rotating magnetic field for around 30 minutes a day, which led to a significant recovery of brain tissue, behaviour, and cognition within 28 days. The ability to stimulate individual cells to repair more neuronal pathways and improve cognitive function could open a number of therapeutic avenues for these graphene nanopatches to be used in different stem cell-based therapies for treating nerve injuries and neurodegenerative diseases.
Reference:
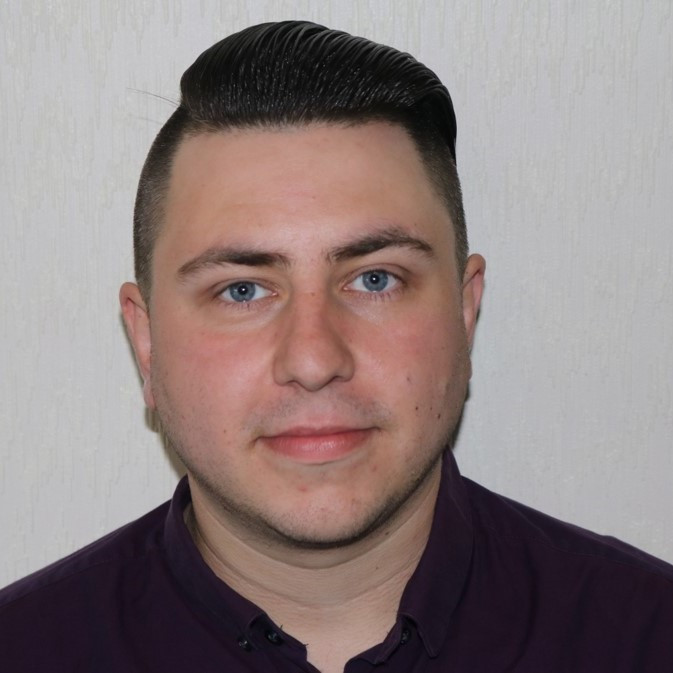