Power Electronics in Smart Grids: Key Role & Future Impact
31-10-2024 | By Robin Mitchell
Table of Contents:
- Introduction
- The Emergence of Smart Grid Technology
- The Role of Power Electronics in Smart Grids
- Why the Electric Grid Needs to Change
- What is a Smart Grid?
- What Advantages do Smart Grids Present?
- What Challenges Do Smart Grids Face?
- How Do Power Electronics Play a Critical Role in Smart Grids?
- What Future Technologies Could Empower Smart Grids?
- Conclusion
Introduction
The energy grid is the most important piece of infrastructure to date, serving as the backbone of modern society for over a century and playing a vital role in our daily lives. The history of power electronics and the grid has shaped how efficiently we distribute power, enabling the complex societies and technological advancements we rely on today. However, despite its importance, the grid has remained largely unchanged since its inception, with only minor tweaks and upgrades over the years.
The Emergence of Smart Grid Technology
The introduction of renewable energy sources and other alternative power sources is now challenging the traditional grid model, and a new concept is starting to gain traction: the smart grid. But what exactly is smart grid technology, and how do power electronics play a crucial role in its implementation?
A smart grid is a power distribution network that utilises advanced technologies, including power electronics, to optimise energy transmission and consumption. This involves the integration of renewable energy sources and the use of advanced metering infrastructure (AMI) for real-time monitoring. The idea behind a smart grid is to create a network that can respond in real-time to changes in energy demand, ensuring that power is delivered efficiently and reliably. This is particularly important for renewable energy sources, which can be intermittent and unpredictable.
The Role of Power Electronics in Smart Grids
Power electronics play a vital role in the implementation of a smart grid, as they enable the efficient conversion of electrical energy between different forms. For example, power electronics can be used to convert DC power from renewable energy sources, such as solar panels and wind turbines, into AC power that can be fed into the grid. Additionally, power electronics can be used to control the flow of power within the grid, ensuring that energy is distributed efficiently and safely.
The use of power electronics in a smart grid also enables the integration of advanced technologies, such as energy storage systems and demand response systems. Energy storage systems can store excess energy generated by renewable sources during periods of high production and release it during periods of high demand. Demand response systems, powered by power electronics, help balance the grid by adjusting energy consumption in real time to match changes in energy supply.
- Efficient Energy Conversion: Power electronics minimise energy losses during conversion processes.
- Enhanced Grid Stability: They support bidirectional power flow and real-time demand response.
As we move forward, the evolution of the energy grid into a smart grid is essential for accommodating the growing demand for energy and the increasing reliance on renewable sources. This transformation not only enhances the resilience and efficiency of our energy infrastructure but also plays a crucial role in addressing climate change and promoting sustainable energy practices. The energy grid, therefore, remains a cornerstone of our society, and its modernisation is vital for a sustainable future.
Why the electric grid needs to change
The electric grid is an intricate infrastructure system that has been the backbone of modern society for over a century. Its ability to distribute power across vast distances and provide a constant supply of electricity empowers all of society, enabling countless technological advancements and fundamentally changing the way we live our lives. Without the grid, we would be forced to return to a bygone era reminiscent of the late 1800s, where energy sources were limited and unreliable, effectively sending us back to the stone age in terms of energy access and technological progress.
For most of its history, the grid has performed admirably due to the predictable nature of electrical demand, the reliance on traditional power stations, and the slow growth in demand. However, the rapid increase in electric vehicle adoption, the shift toward renewable energy sources, and the sudden changes in energy demand are placing extraordinary stress on the grid. The rise of decentralised energy systems further complicates grid management. While some degree of intelligence has been integrated into the system, it is still struggling to cope with the changing landscape.
Challenges in Grid Stability and Management
One of the primary challenges facing the grid is the increasing demand for energy during peak hours. As more households switch to electric heating and vehicles, the demand for electricity during these periods surges, putting pressure on power stations to meet the demand. However, the integration of renewable energy sources, such as solar and wind, introduces a new level of complexity, as their output is often unpredictable and intermittent. This variability makes it challenging for grid operators to balance supply and demand, leading to potential power outages and brownouts.
Another crucial challenge facing the grid is the need for greater flexibility and adaptability. The increasing demand for energy storage solutions, such as batteries, is evidence of the grid's inability to store excess energy generated by renewables during off-peak hours. This lack of flexibility not only hampers the grid's ability to respond to sudden changes in demand but also makes it difficult to integrate new energy sources, such as hydrogen and biogas, which require specific storage and handling conditions.
Environmental and Social Impacts
In addition to these technical challenges, the grid also faces pressing social and environmental concerns. The increasing demand for renewable energy sources has led to the construction of large-scale wind farms and solar parks, which can have devastating impacts on local ecosystems and wildlife habitats. The need for energy storage solutions has also led to the development of new technologies, such as pumped hydro storage, which can have considerable environmental impacts if not managed properly.
What is a smart grid?
The concept of a smart grid is not entirely new; however, the technology surrounding it is rapidly advancing, making it increasingly significant in the electrical engineering field. A smart grid is essentially an electrical grid enhanced by advanced technologies, such as IoT, aimed at improving efficiency and reliability.
In contrast to traditional electrical grids, a smart grid is a dynamic system capable of responding to real-time changes. For example, smart meters and advanced metering infrastructure (AMI) are central to this heightened responsiveness, serving as intelligent devices that monitor and control electricity flow. These meters not only track the amount of electricity consumed by households or businesses but also relay this data back to grid operators in real-time. This information is utilised for demand forecasting and supply adjustments, ensuring optimal grid operation.
Smart Grid concept illustration showcasing renewable energy architectures, smart energy networks, smart city applications, and the Internet of Things.
Benefits of Smart Grids for Consumers
One of the most remarkable benefits of a smart grid is its capacity to offer variable tariffs to customers. By monitoring real-time demand, grid operators can pinpoint periods of low usage and provide discounted rates during these times. This approach not only reduces overall electricity costs for consumers but also encourages them to modify their energy consumption patterns to take advantage of these lower rates.
For instance, a customer away from home during the day might benefit from a reduced tariff by scheduling appliances like washing machines or dishwashers to operate during this time. This can then be taken further, whereby connected devices can sleep based on live tariff rates, further optimising energy usage and cost savings. For example, a connected electric vehicle (EV) could prevent charging during peak times when electricity rates are high and then start charging when rates drop below a defined level, maximising cost efficiency for the owner.
Decentralised Energy Systems and Microgrids
The application of smart grids extends beyond mere energy consumption. Connected devices such as smart thermostats and electric vehicles can be integrated into the grid to contribute additional energy sources. These devices can be programmed to pull power from the grid during low demand and return energy during high demand periods, helping to stabilise the grid while offering customers a new revenue stream and promoting sustainable, cost-effective energy usage.
What advantages do smart grids present?
Smart grids have been touted as the future of energy distribution for several years, and it is easy to see why. One of the biggest advantages of smart grids is that they are able to make the most of renewable energy sources. This is achieved by utilising devices connected to the grid, which can be used to store energy during times of peak generation and then feed this back into the grid when demand is at its highest. By doing so, the amount of energy that is wasted is significantly reduced, which can have a major impact on the environment.
Reducing Reliance on Fossil Fuels
Increasing the amount of energy extracted from renewable sources not only makes them more economical but also helps to reduce our reliance on fossil fuels. Fossil fuels are not only problematic for the environment, but they also present socio-political challenges, which is why many countries around the world are trying to move away from them.
Smart grids can also help consumers to be more energy efficient, thereby reducing CO2 production. This can be achieved in various ways, including the use of smart meters that can detect when energy is being wasted and smart home systems that can automatically turn off devices that are not in use.
Stabilising Energy Consumption
Another advantage of smart grids is that they can be used to help stabilise consumption, which can be achieved with the use of variable tariffs. These tariffs consider the time of day and the amount of energy being generated, encouraging consumers to use energy when it is being produced. This not only helps to reduce energy waste but also lowers the amount of CO2 being produced by the grid.
Finally, the use of smart grids can also help to reduce the need for grid operators to store power and/or fire up backup systems to provide grid stability. This is especially helpful in emergency situations where power to critical infrastructure is needed, yet powerlines or power stations are damaged.
What challenges do smart grids face?
The reality of implementing such a system is far more complex than many may realise. While the idea of a smart grid sounds great, it is far from trivial to implement, and there are a number of challenges that must be overcome before such a system can become a reality.
One of the biggest challenges faced by smart grids is the need for connected technologies that can work together seamlessly. A smart grid is only as smart as its weakest link, and if any one technology in the system fails, the entire grid can be compromised. This means that all connected devices, from energy meters to power storage systems, must be able to communicate with each other and make decisions based on real-time data. This level of interconnectivity is no small feat, and the need for all devices to be able to communicate with each other in real time makes it even more challenging.
Safety and Power Electronics Challenges
Another challenge faced by smart grids is the need for connected devices to be able to safely push and pull power to and from the grid. Traditional power systems have a clear separation between the grid and individual devices, with only a small amount of power being fed back into the grid from individual devices. However, in a smart grid, devices are expected to be able to draw and push large amounts of power at short notice, making safety a major concern. This means that not only must devices be able to handle large amounts of power, but they must also be able to do so in a way that is safe for both the device and the grid as a whole.
The power electronics involved with smart grid systems is also far from trivial. For example, connected electric vehicles need to have the ability to charge and discharge their batteries at short notice, and this requires the use of high-power electronics. The complexity of these electronics is only increased when considering that they must also be able to communicate with the grid and other devices in real-time. The need for high-power electronics also makes the design of smart grid systems more expensive, as the cost of high-power components can be prohibitively expensive.
Consumer Adoption and Infrastructure Upgrades
Negotiating live tariffs with energy providers is also a major challenge faced by smart grids. The vast number of different energy sources available to consumers, including solar and wind, makes it difficult for any system to determine what rates should be charged. If not done correctly, it is possible for grids to become overloaded, which can have serious consequences for the stability of the grid. Any live response from smart systems must be over minutes, not seconds; otherwise, feedback loops can occur which are difficult to control.
Getting consumers to switch to smart technologies is also a major challenge faced by smart grids. If consumers do not see the benefits of switching to a smart grid, they may be unwilling to do so, and this can make it difficult for a smart grid to become a reality. Furthermore, replacing older hardware can be a major challenge, especially if it is no longer supported by the manufacturer. This can make it difficult for a smart grid to be implemented, as older hardware may be required for the system to function properly.
How do power electronics play a critical role in smart grids?
The world is swiftly moving towards a new era of smart technologies, and nowhere is this more evident than in the field of smart grids. The integration of power electronics into these systems is crucial for their operation, but understanding the various applications of power electronics in smart grids can help engineers develop the latest power systems more effectively.
One of the primary applications of power electronics in smart grids is bi-directional power systems. These systems will play a critical role in smart grid systems, as they will allow for the efficient distribution of energy between power sources and consumers. Bi-directional power systems will be particularly useful for electric vehicles (EVs), which can act as virtual batteries during times of high energy production. Bidirectional power flow enables this functionality. Chargers for EVs need to be able to both charge the vehicle and return that power back into the grid when necessary. As a result, charger circuits must be equipped to handle large voltages and currents, withstand transients, and operate safely. SiC and GaN technologies enhance these capabilities.
Power Monitoring and Control in Smart Grids
In addition to bi-directional power systems, power electronics are also essential for power monitoring in smart grids. Any system connected to a smart grid must be able to monitor its power consumption, which relies on the use of voltage and current monitoring. This data must also be communicated to grid operators, who can then make informed decisions based on consumption patterns. In some cases, there may even be a need for reverse control from the grid, allowing devices to be put to sleep during times of peak consumption.
Infrastructure and Communication Technologies
Another critical aspect of smart grids is the infrastructure needed to support them. Smart grids will be heavily dependent on Internet infrastructure, which must be both reliable and easily accessible. Not all smart grid devices will have access to local networks, meaning that they may require long-range options such as cellular and LoRa. Furthermore, manufacturers of power systems connected to the grid will need APIs and other internet-enabled technologies to connect their designs to grid operators.
What future technologies could empower smart grids?
The future of smart grids is set to be greatly influenced by advancements in state-of-the-art technologies that will enhance the efficiency, reliability, and sustainability of energy distribution. Among the numerous emerging technologies, silicon carbide (SiC) and gallium nitride (GaN) stand out as promising solutions, ready to reshape the landscape of smart grids.
SiC is particularly well-suited for high-voltage applications, enabling devices to operate at greater voltages than traditional silicon-based technologies. This capability allows for the development of power systems that can handle higher voltages while improving efficiency through reduced current losses. Operating at elevated voltages also results in increased power densities, making SiC an attractive choice for applications requiring compact size and space efficiency.
In contrast, GaN excels in low-voltage applications, offering significant advantages over SiC. Although GaN may not support as high voltages as SiC, it facilitates the creation of extremely small power circuits that are more compact compared to those based on SiC. In applications where space and size are a virtue, GaN can exceed SiC, leading to substantial energy savings, as smaller devices typically consume less energy. When applied to renewable energy sources, GaN can significantly enhance the efficiency of low-voltage devices, further reducing overall power consumption.
Bidirectional Power Flow and Advanced Monitoring
The integration of SiC and GaN technologies into smart grids will profoundly impact energy distribution's future. A key benefit of these technologies is the potential for bidirectional power flow. Traditional power grids operate unidirectionally, with power flowing from plants to consumers. However, incorporating SiC and GaN technologies will enable bidirectional power systems, allowing energy to flow in both directions. This functionality supports the integration of renewable energy sources and facilitates the development of microgrids, which can operate independently from the main grid.
Another substantial advantage of SiC and GaN technologies is the possibility of advanced power monitoring and control systems. The enhanced efficiency and power density from these technologies will allow for smaller, more sophisticated power monitoring devices capable of measuring power consumption in real time. Insights gained from this data will help optimise energy distribution, reduce waste, and minimise the environmental impact of energy production.
Furthermore, the integration of SiC and GaN technologies will enable intelligent control systems that analyse real-time data from power monitoring devices to optimise energy distribution and consumption. By leveraging advanced algorithms and machine learning techniques, these systems can identify patterns in energy usage and make data-driven decisions to reduce energy waste and maximise efficiency.
Additional Technologies Enhancing Smart Grids
In addition to SiC and GaN, other future technologies could further empower smart grids. For instance, energy storage solutions, such as advanced battery technologies and supercapacitors, will play a crucial role in balancing supply and demand, especially with the increasing reliance on intermittent renewable energy sources. Additionally, the Internet of Things (IoT) can enhance connectivity and communication between devices, allowing for more responsive and adaptive energy management systems. Blockchain technology may also provide secure and transparent transactions for energy trading among consumers and producers, fostering a decentralised energy market.
Conclusion
Smart grids promise to revolutionise how we generate, distribute, and consume electricity, enhancing efficiency and reliability while integrating renewable energy sources. Smart grid technology is essential for climate change mitigation. The need for power electronics in such grids is essential, as they facilitate the control and conversion of electrical energy, enabling the seamless integration of diverse energy sources and improving the overall performance of the grid.
Technologies such as Silicon Carbide (SiC) and Gallium Nitride (GaN) are particularly promising in this context. These wide-bandgap semiconductors offer higher efficiency, greater thermal performance, and the ability to operate at higher voltages and frequencies compared to traditional silicon-based technologies. Their integration into power electronics can significantly enhance the capabilities of smart grids, allowing for more efficient energy conversion and management.
Power electronics play a critical role in managing the flow of electricity, ensuring stability, and optimising energy usage, which is vital as we transition to a more decentralised and renewable energy landscape.
The use of bidirectional power will help maintain power demand, but it will be tricky to correctly deploy at scale. This technology allows for energy to flow in both directions, enabling not only the consumption of electricity but also the return of excess energy back to the grid. This capability is particularly important in the context of renewable energy sources, which can be intermittent.
By effectively managing this bidirectional flow, we can enhance grid stability and ensure that the energy supply meets demand more efficiently.
As we move forward, it will be essential to monitor advancements in this area, particularly the role of SiC and GaN technologies, as they will play a critical role in shaping our energy landscape. Adopting these technologies promotes sustainable energy practices. Their ability to improve efficiency and reduce losses in power conversion will be vital for the successful implementation of smart grids.
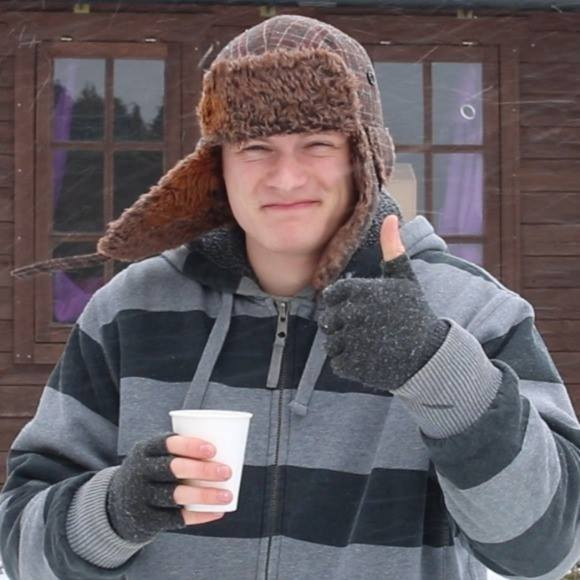