Nanofluidic Chemoelectrical Generator for Scalable Energy Use
27-11-2024 | By Liam Critchley
The need for power at all device levels is increasing. While there is often a focus on developing large-scale energy storage and harvesting devices to meet larger energy demands, there has also been a lot of growth in small-scale energy storage and harvesting devices using nanotechnology. There are many small, different, small-scale energy harvesters, with nanogenerators being the most common devices to date. This has often focused around triboelectric nanogenerators (TENGs) and piezoelectric nanogenerators (PENGs), but a recent study has looked at how a nanofluidic chemoelectrical generator (NCEG) can be used to harvest energy.
Key Things to Know:
- Breakthrough in Small-Scale Energy Harvesting: Nanofluidic chemoelectrical generators (NCEGs) harness the Coulomb drag effect to amplify energy output, enabling efficient power generation without external electrical fields.
- Innovative CNT Membrane Design: Advanced carbon nanotube (CNT) membranes optimise ionic flow and reduce resistive losses, delivering milliampere-level currents and scalable performance up to tens of volts.
- Integration of Hybrid Materials: Combining materials like aluminium and gold enhances system durability and stabilises ionic flows, ensuring consistent energy conversion in diverse environmental conditions.
- Future Applications and Research: NCEGs show potential for medical diagnostics, portable energy solutions, and autonomous systems, with ongoing research exploring 2D materials and adaptive machine-learning-driven configurations.
Coulomb Drag Seen in Nanofluidic Devices
The phenomenon of Coulomb drag was first seen in electron-electron interactions where electrically isolated conductor layers are located close together. In these conductor layers there is an active and passive layer due to the long-range interactions that occur. When the electrical current flows through one conductive layer, it becomes the active layer, and it then induces an electrical current in the passive layer.
Throughout this process, the energy and momentum of the charge carriers pass from the active layer to the passive layer, which generates and open circuit voltage and a short-circuit current because the charge carriers are ‘dragged’ along. The generated current in the passive layer is driven completely by Coulombic interactions, and this phenomenon has also been seen in nanofluidic devices. This discovery showed that Coulomb drag can be extended to a moving ionic fluid and electrons in conductors.
It has been shown that graphene can generate a nanoampere current using an ionic flow by applying a voltage to a nanofluidic chamber. This approach has shown that fluidic flows can induce an electrical polarization to drive electrical currents. It has also been shown that an externally biased voltage can generate an electrical current in single-walled carbon nanotube (SWCNT) layers because the electrons get dragged through the SWCNT nanochannels. This approach has created ionic drag currents because the confined ion-electron interactions cause a nanofluidic Coulomb drag. In SWCNT nanochannels, the ionic current can be amplified due to the difference between the ion mass and hole mass on the membrane.
There is still more work to be done regarding the amplification of an ionic current in these membranes, as the studies to date required an external electrical field to drive the electrons and ionic flow. However, the need for an external electrical field makes devices a lot more complex and less practical for real-world use, so there’s an interest in seeing if this Coulombic drag can amplify a current in SWCNT devices without the external field.
New NCEG Based on a CNT Membrane
Recent studies suggest that utilising advanced CNT membrane configurations can further enhance the current output by optimising the porosity and conductivity of the nanochannels. The introduction of nitrogen-doped CNTs has been shown to reduce resistive losses significantly, enabling higher efficiency in energy transfer across the electrodes.
Researchers have now developed a self-driving NCEG using a CNT membrane that is constructed by sandwiching a 25 mm2 CNT membrane between two metal electrodes. The device managed to deliver a milliampere-level current output.
To better understand the design and functionality of the NCEG, the schematic representation below illustrates its key components and operation. The carbon nanotube membrane, sandwiched between two electrodes, facilitates ionic flow driven by spontaneous redox reactions. This unique configuration enables the generation of milliampere-level currents, demonstrating the device's advanced energy harvesting capabilities.
Figure 1: a The structural illustration of the NCEG: A porous carbon nanotube membrane (CNTM) with a highly ordered array is positioned between two metal electrodes, consisting of gold foil and conductive carbon tape with an aluminium substrate. When the electrolyte solution is applied to one side of the CNTM and partially fills the nanochannels, a redox potential is generated, driving ionic movement within the nanochannels and amplifying the circuit's current through the ion-electron Coulomb drag effect. b Morphological features of the CNTM and X-ray photoelectron spectroscopy (XPS) analysis of both the pristine anodic aluminium oxide (AAO) membrane and the CNTM. c Diagram illustrating the ionic Coulomb drag effect, depicting ionic movement along the CNTM and the corresponding dragging of free electrons within the CNTM. d Open-circuit voltage and short-circuit current density produced by the NCEG in a 0.1 M NaCl electrolyte.
Self-Sustaining Ionic Flow through Metal-Air Redox Reactions
The ionic flow in the NCEG was driven by itself using metal-air redox reactions (between metal and oxygen) to convert chemical energy into electrical energy without the need for an external electrical field. These redox reactions are spontaneous and establish a chemical potential across the membrane that drives the migration of ion in the nanochannels of the membrane.
Additionally, the integration of hybrid electrode materials, such as aluminium and gold, into the NCEG framework has demonstrated potential for improving the durability of the system under prolonged operational conditions. By leveraging the redox properties of these materials, researchers aim to enhance the chemical potential consistency, further stabilising ionic flow within the nanochannels.
Amplifying Current with the Coulomb Drag Effect
The Coulomb drag effect was realised in the NCEG by moving ions and electrons within the CNT membrane, which led to the current coupling of the ion-electron becoming amplified—16 times higher than the current collected without the CNT membrane. The device generated a sustained direct voltage of ~0.8 V and a short-circuit current density of ~1.2 mA cm-2, with a power density of 74 μW cm-2 for 80 hours. The device also showed a linear scalable performance up to tens of volts.
Experimental observations also revealed that the device's performance remains unaffected by minor fluctuations in ambient conditions, such as temperature and humidity, highlighting its robustness. This makes the NCEG a promising candidate for deployment in diverse environmental settings, ranging from industrial applications to portable energy solutions.
The work by the research team is the first experimental evidence that ion-electron Coulomb drag effects can amplify currents for energy harvesting applications. While this is the first device of its kind, there are many directions that future research can go in.
Integrating 2D Materials for Multi-Energy Harvesting
The researchers have stated that they are going to be looking at different nanomaterial membranes to facilitate self-driven Coulomb drag amplification. These include 2D material membranes with a photoelectric or thermoelectric response made from graphene and molybdenum disulphide materials.
Exploring the potential of 2D materials like graphene oxide and molybdenum disulphide, researchers are investigating methods to amplify photoelectric and thermoelectric responses. These materials are known for their exceptional conductivity and low thermal losses, which could pave the way for hybrid energy harvesting systems capable of integrating solar and thermal energy sources.
The researchers have also stated that they are looking into mechanisms for activating the energy in the system, as this will be key for the devices having the ability to harvest energy from multiple sources. Some mechanisms that the researchers will be looking into include ion interactions and exposing electrons to light and thermal stimulation. It’s also been stated that further work will need to be made to the NCEG if it is to be used as a power source for different applications, including medical diagnostics and emergency communications systems, but it’s the first step in the direction towards creating a new class of small-scale energy harvesters.
Towards a New Generation of Energy Harvesters
Future advancements in NCEG design could incorporate machine learning algorithms to dynamically optimise device configurations based on real-time data. Such innovations could allow for adaptive performance tuning, ensuring maximum energy efficiency across varying operational scenarios, from wearable medical diagnostics to grid-independent emergency communication systems.
Conclusion
The development of nanofluidic chemoelectrical generators (NCEGs) represents a significant breakthrough in the field of energy harvesting, offering a novel approach to harnessing energy at small scales. By leveraging advanced materials such as carbon nanotube membranes and exploiting the Coulomb drag effect, these devices provide a scalable and efficient solution for generating power in a wide range of applications. The self-driving capability of NCEGs, driven by spontaneous redox reactions, eliminates the need for external energy inputs, simplifying their design and expanding their potential uses.
With promising advancements, such as the incorporation of hybrid nanomaterials and enhanced electrode configurations, researchers are paving the way for even more robust and versatile energy harvesters. Future iterations of NCEGs could address the growing demands of portable power solutions, contributing to areas such as medical diagnostics, emergency communication systems, and autonomous sensing devices.
As this technology matures, integrating multi-energy harvesting mechanisms and adaptive performance enhancements could further unlock its potential. The exploration of photoelectric, thermoelectric, and machine-learning-based optimisations will likely expand the scope of NCEG applications, making them indispensable in modern energy solutions. Ultimately, NCEGs stand at the forefront of innovation, shaping the future of sustainable, small-scale energy harvesting systems.
References:
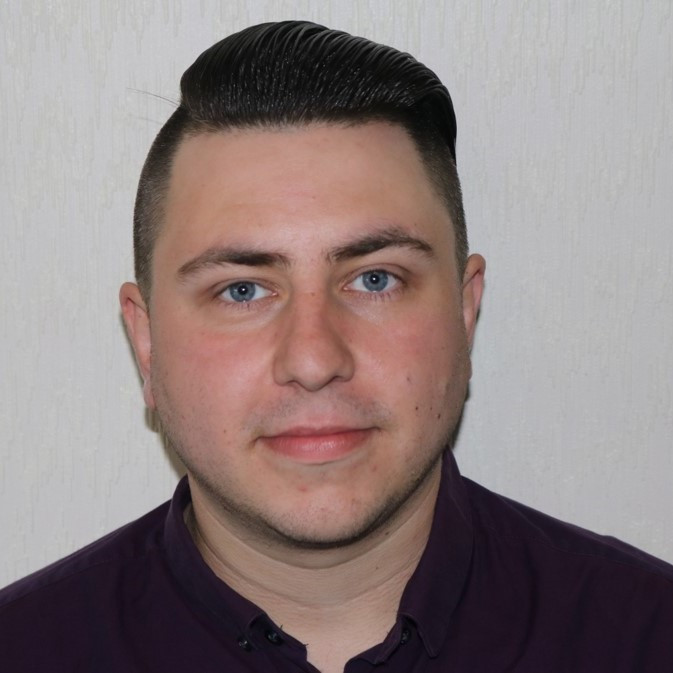