Writing with Atoms: Transforming Quantum Device Fabrication
05-11-2024 | By Robin Mitchell
An artistic rendering illustrates a method for building materials atom-by-atom. Here, an electron beam removes a carbon atom from graphene, allowing a different atom to bond precisely at the vacancy. Credit: Ondrej Dyck/ORNL, U.S. Department of Energy.
Recently, researchers demonstrated a new technique for manipulating the position of atoms to create new devices and materials on a quantum scale. What challenges does nanodesign face, what exactly did the researchers achieve, and how could it help the future of sensing in electronics.
Key Things to Know:
- Atomic-Scale Precision: A pioneering tool called the synthescope now allows researchers to manipulate individual atoms, enabling the creation of materials with highly specific properties crucial for advancements in quantum computing and sensing.
- Overcoming Quantum Stability Challenges: This technology stabilises qubits, essential elements in quantum computing, by placing atoms precisely to maintain quantum coherence, directly addressing one of the major hurdles in scalable quantum technology.
- Beyond Quantum Computing: The synthescope’s applications extend to microelectronics, catalysis, and advanced material synthesis, supporting innovations in miniaturised electronics and next-generation materials science.
- Implications for Advanced Sensing: With the potential to detect individual photons or electrons, this tool could revolutionise fields such as secure communications, medical diagnostics, and even environmental monitoring, making ultra-sensitive quantum sensors a reality.
The Challenges of Modern Nanodesign
In the field of technological development, human ambition has historically oscillated between the construction of monumental structures and the meticulous crafting of minuscule devices. This dual fascination is vividly illustrated in the evolution of semiconductors, a field where the push towards miniaturization represents not merely a desire for compactness, but a relentless quest for enhancing performance, efficiency, and functionality.
Moore’s Law and the Push Towards Smaller Transistors
The semiconductor industry, a cornerstone of modern technology, has been driven by an overarching principle known as Moore's Law, which posits that the number of transistors on a microchip doubles approximately every two years, though this pace has slowed in recent years. This exponential growth is made possible by the consistent reduction in the size of each transistor, allowing more of them to be packed into a single chip. The benefits of this scaling are manifold: as transistors shrink, the distances that electrons need to travel decrease, which typically increases the speed and energy efficiency of devices.
However, the pursuit of ever-smaller transistors confronts fundamental physical limits. One such limit is the size of the atoms themselves. In traditional semiconductor design, a transistor cannot be smaller than the size of an individual atom. Despite this, the industry's approach has been to edge ever closer to these atomic boundaries, driven by innovations in techniques such as extreme ultraviolet (EUV) lithography.
Extreme Ultraviolet (EUV) Lithography and Its Constraints
EUV lithography is a sophisticated method that uses extremely short wavelengths of light to etch minuscule patterns on silicon wafers, enabling the production of smaller features than what was previously possible with older lithography techniques. Despite its advancements, EUV lithography still faces significant challenges. The wavelength of light, albeit much shorter than that used in traditional lithography, still imposes a limit on the minimum feature size that can be reliably produced. Additionally, the complexities of chemical processing further constrain the attainable resolutions.
Moreover, while techniques exist for placing individual atoms with precision, scaling this process to the manufacturing levels required for modern semiconductor devices is currently impractical. The construction of devices with billions of transistors using atomic-scale placement would be an exceedingly slow and, thus, economically unfeasible process.
Exploring Alternatives and New Materials
Given these constraints, researchers in the field of semiconductors are continuously exploring alternative methods and new materials to circumvent the limitations imposed by current technologies. Some of these innovative approaches include the use of two-dimensional materials like graphene, which might allow for the creation of transistors at scales smaller than currently possible with silicon-based technologies. Additionally, advancements in quantum computing offer a paradigm shift in how information is processed, potentially bypassing some of the limitations of traditional transistor-based technologies.
The drive towards atomic-scale semiconductor devices also raises important considerations regarding energy consumption and heat dissipation. As components shrink, the power density increases, leading to greater challenges in managing heat within the device. Innovative cooling techniques and materials that exhibit superior thermal properties are, therefore, integral to the continued progress in semiconductor miniaturization.
Researchers Develop New Mechanism for Individual Atom Manipulation
In a groundbreaking development at the Department of Energy's Oak Ridge National Laboratory, a team of scientists has pioneered a new technology that could transform the field of quantum computing and communication. The technology, which involves a novel advanced microscopy tool called a synthescope, allows for the precise placement of individual atoms within materials, potentially giving rise to entirely new properties and capabilities.
The recent advancements at Oak Ridge National Laboratory (ORNL) have led to the creation of the synthescope, a groundbreaking tool that enables precise atomic manipulation. This technology allows scientists to position individual atoms with unprecedented accuracy, a capability that promises to revolutionise fields such as quantum computing and materials science. By transforming a scanning transmission electron microscope, the synthescope introduces new possibilities for developing advanced materials and quantum devices with tailored properties.
An illustration of the heater platform used in the synthescope, which supplies atomised material to samples, transforming a scanning transmission electron microscope into a tool for precise atomic manipulation. Credit: Ondrej Dyck/ORNL, U.S. Department of Energy
Beyond theoretical advancements, this precise atom placement has implications for real-world applications in quantum computing, specifically by stabilising qubits. By introducing atoms with specific properties at exact locations, the synthescope enables greater control over quantum states essential for quantum information processing. This method aligns well with the unique demands of quantum technologies, which require extremely delicate configurations to maintain quantum coherence and reduce error rates in computations.
Combining Synthesis and Advanced Microscopy
The synthescope combines synthesis with advanced microscopy and utilises a scanning transmission electron microscope transformed into an atomic-scale material manipulation platform. This innovative tool enables researchers to insert different atoms into a material at specific locations, which can be strategically chosen to enhance the material's properties.
The precision of the synthescope holds the potential to fundamentally transform materials engineering, particularly for applications requiring atomic accuracy, such as microelectronics and next-generation quantum devices. Researchers can achieve unprecedented accuracy in the placement of atoms to create materials with enhanced electronic, optical, and thermal properties, which are crucial for improving device performance in various high-tech fields.
Working at the Quantum Scale: The Power of Precision
Leading the research, Stephen Jesse, a materials scientist at ORNL's Center for Nanophase Materials Sciences, emphasised the significance of working at the atomic scale. He noted, "By working at the atomic scale, we also work at the scale where quantum properties naturally emerge and persist." Jesse further explained that this access to quantum behaviour lays a foundational stone for future devices that leverage quantum phenomena like entanglement to improve computers, enhance secure communications, and increase the sensitivity of detectors.
Working at the atomic scale also provides opportunities for innovations in quantum sensors, which benefit from enhanced sensitivity to detect minute physical changes. The synthescope’s capability to embed atoms precisely enables the construction of ultra-sensitive detectors for both photons and electrons, paving the way for advancements in areas like secure communication, cryptography, and even medical diagnostics by allowing detection of molecular-level interactions.
Enhancing Quantum Computing Stability
The technology's potential extends beyond just theoretical applications. Ondrej Dyck, another materials scientist involved in the project, highlighted its practical implications for quantum computing. Quantum computers, which use qubits capable of existing in multiple states simultaneously, could greatly benefit from this technology as it addresses the challenge of stabilising these qubits. By manipulating materials at the atomic level, where quantum mechanics are more naturally observable, researchers can more effectively maintain the stability and functionality of qubits.
This atomic-level manipulation addresses a key challenge in quantum computing: maintaining qubit stability amidst environmental disturbances. By positioning atoms that enhance qubit coherence, the synthescope aids in achieving stable quantum states that are necessary for performing consistent computations, a significant leap in overcoming one of the primary barriers to scalable quantum technology.
Applications Beyond Quantum Computing: Catalysis and Microelectronics
The ability to tailor materials atom-by-atom not only benefits quantum information science but also has implications for microelectronics, catalysis, and material synthesis processes. This could lead to advances in atomic-scale manufacturing, a field that has been notoriously difficult to master.
Atom-by-atom fabrication could lead to breakthroughs in miniaturised electronics, allowing for devices that operate with greater efficiency and reduced power consumption. The approach supports not only innovations in quantum computing but also opens avenues in catalysis and microelectromechanical systems (MEMS) where precision-engineered materials could enable highly specific chemical and physical reactions.
Unlocking Quantum Entanglement for Advanced Devices
Moreover, the technology opens up possibilities for creating arrays of precisely positioned atoms that can share quantum properties through entanglement, a key feature for enhancing the power of quantum devices. These devices could include next-generation quantum computers that surpass today's fastest supercomputers, as well as quantum sensors and secure quantum communication systems that require single-photon sources.
Moreover, by manipulating individual atoms to create entangled states, scientists can develop quantum devices that provide unparalleled security in data transmission. This atomic control can also lead to sensors that detect single photons or electrons, advancing fields such as ultra-sensitive environmental monitoring, biological imaging, and astrophysics, where detecting individual particles can offer insights previously unattainable.
Future Ambitions and Impact of Atomic Fabrication
Summing up the project's ambitions, Jesse stated, "We are not just moving atoms around. We show that we can add a variety of atoms to a material that were not previously there and put them where we want them. Currently, there is no technology that allows you to place different elements exactly where you want to place them and have the right bonding and structure."
The synthescope thus represents a leap forward in atomic fabrication, moving beyond existing techniques by integrating atomic positioning with atomic bonding. This innovation is set to enhance not only the structural integrity of new materials but also their functional properties, offering tailored solutions for a range of applications, from robust quantum devices to high-performance electronic components.
Could We Ever See Single Atom Devices?
Despite these advancements, the direct translation of this technology to large-scale production remains elusive. The primary obstacle is the sheer complexity and the level of precision required to scale up from a single atom to millions or even billions, which are necessary for practical devices. Each atom must be meticulously placed, and even minor inaccuracies can lead to significant deviations in performance at larger scales.
However, it is in the field of advanced sensing, particularly in quantum devices, where this technology harbors the potential to be transformative. By harnessing the ability to manipulate devices at the atomic level, it becomes possible to create sensors that operate with unprecedented sensitivity and specificity. These sensors could, for instance, detect and measure individual electrons and photons, a capability that is crucial for advancing our understanding of quantum mechanics and for developing new quantum information technologies.
Imagine laboratories equipped with sensors tailored for specific research tasks capable of detecting minute quantum phenomena that were previously undetectable. Such sensors could lead to the development of new materials with novel properties, more efficient energy systems, or even entirely new modes of computation. The potential applications are vast and could significantly accelerate scientific research and technological innovation.
Moreover, the sensitivity of these devices could unlock new insights into the physical and quantum world. For instance, researchers could observe and manipulate quantum effects in real-time at unprecedented scales, potentially leading to breakthroughs in quantum computing, cryptography, and teleportation. The implications of such capabilities stretch far beyond the laboratories and could redefine the technological landscape of the future.
In conclusion
While the scale-up of single atom devices to larger, practical systems remains a formidable challenge, the potential applications in the field of advanced sensing and quantum devices are profound. The ability to control matter at the atomic level could herald a new era of technology, marked by an enhanced understanding of the quantum realm and significant advancements in a multitude of scientific disciplines. The journey from theoretical possibility to practical application is fraught with technical hurdles, but the rewards promise to be of a scale that could reshape our technological world.
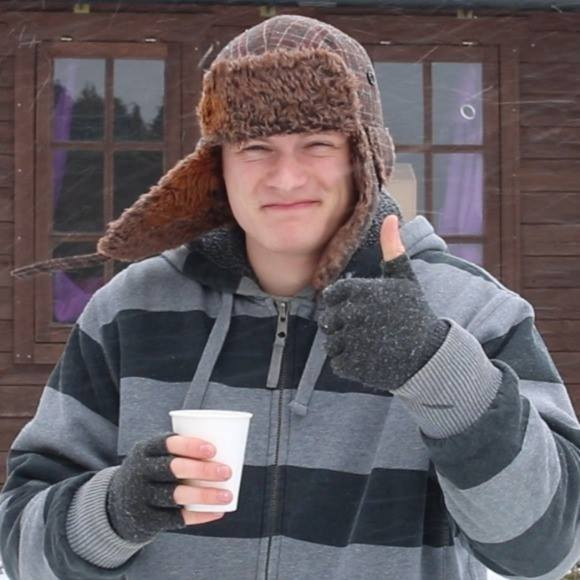