Microfluidic Sensors for Lab-On-A-Chip Technologies
02-01-2025 | By Gary Elinoff
Image source: Alineinc
Microfluidic Sensors for Lab-On-A-Chip Technologies:
- There are many parallels between digital computer chips and Microfluidic Labs-On-A-Chip.
- Microfluidic Chips are composed of microscopically thin channels connecting an array of tiny valves, mixers and pumps.
- These Labs-On-A-Chips have many applications in drug development, food safety, disease detection and more.
Introduction
As described by Ossila[1], microfluidics (MF) is "field of research focused on the development of fluid-processing technology at the microscale. Imagine a circuit board, but for liquids: microfluidic devices, often referred to as "chips", are patterned with minuscule channels and ducts through which liquids flow. In these compact and constricted conditions, liquid exhibits unique behaviours that can be harnessed for rapid and high-throughput results."
In a further similarity to semiconductor technology, microfluidics also depends on photolithography, which "involves transferring geometric shapes from a mask onto a substrate, using special polymers that react to specific wavelengths of light. This process creates the intricate patterns necessary for microfluidic devices." Micro Electro Mechanical Systems (MEMS) is a key technology employed in photolithography. This fascinating technology is described at length in Jack Pollard's informative article What are MEMS? A Comprehensive Guide to Microelectromechanical Systems.
While semiconductor chips are purposed for the various digital and analog functions necessary for modern electronic systems, microfluidic chips, as described by Alineinc[2], "typically consist of a network of tiny channels, valves, and pumps that can control fluid flow and mixing at the microscale level." These devices can be thought of as mini chemistry labs on a "chip", with the microfluidic sensors playing the starring role. These sensors serve to measure vital chemical parameters such as pH and temperature.
About Microfluidic Sensors
Microfluidic devices incorporate a veritable maze of microscopic channels that connect to external fluid sources. Flow is controlled by equally tiny devices such as valves, mixers, and pumps, achieving the same sort of results as large-scale, human-operated laboratories. One of the main selling points is the tiny amounts of samples and reagents required, often measured in microliters or even picoliters.
Microfluidic (MF) sensors make Labs-On-A-Chips (LOC) possible. As described by AZO Sensors[3], important use cases include:
- Infectious disease diagnostics
- Cancer diagnostics
- Chronic disease management
- Neonatal and prenatal care
- Environmental and food safety monitoring
- Drug development
These tiny LOCs incorporate detection modules to detect biochemical interactions. Electrochemical sensors detect changes resulting from biochemical reactions, enabling them to measure glucose and lactate, while optical sensors monitor molecular interactions. Additionally, piezoelectric sensors detect mass changes associated with biomolecular binding events.
Actuators and valves are employed to effect changes within microfluidic devices, such as regulating the flows and mixing of reagents and test samples. Real time communication to the actuators and sensors enable control and monitoring.
As pointed out by AZO Sensors, the ability to communicate directly with patients' smartphones has made these devices a powerful modality on which to base home-based infectious disease monitoring. In the following sections, we'll explore some of the other uses for microfluidic devices.
What Goes on Inside a Microfluidic Sensor?
As an example, we examine Cellix's [4] Microfluidic Flow Sensor, which works on the principle of thermal mass flow measurement.
Image source: We are Cellix
There is a microchip (green) controlling two tiny temperature sensors, one on each side of an equally minuscule heating element (red). The temperature sensors and the heater are placed at the exterior of the flow channel, thereby physically but not thermally isolating them from the tiny liquid flow.
The microchip's input includes the amount of heat that the heater infuses, as well as the upstream and downstream temperature of the traversing liquid. It is from these inputs that the microchip calculates the volume of flow through the microfluidic channel that the microfluidic sensor is measuring.
Food Safety
Food can be infected with pathogens at any stage of its production, transportation or final preparation, making expensive, time consuming centralized testing unworkable and impractical. As noted by Elveflow[5], this creates a need for inexpensive, in situ testing that can match the accuracy of standard gas chromatography-based mass spectrometry.
Microfluidic paper-based analytical devices (µPAD), owing to their low cost, rapid response and excellent biocompatibility, are fast emerging as preferred responses. These devices can accomplish the necessary pretreatment, sensing and unambiguous output signal generation.
Human Cell Cultures through Lab-On-A-Chip Technology
Microfluidic based LOCs can now be used to micro-engineer single or multi-cell cultures, effectively modeling living human cells and organs. One of the manifestations of this ability is the creation of what can only be described as organs on a chip (OOC).
As described in an article by Biotium[6], researchers have "generated human pancreatic islets and liver spheroids on-chip. This two-organ-chip model, complete with a working feedback loop between the liver spheroids and the insulin-secreting islet microtissues, gave the researchers the ability to study pancreatic islet–liver cross-talk based on insulin and glucose regulation."
Image source: Bauer though Biotium
The left panel of the image above illustrates a two organ LOC. The panel on the right shows pancreatic islet microtissues and liver spheroids stained for insulin(red) and glucagon (green).
Being able to create very specific, living (in vivo) combinations of human organs will make "the real thing", an actual human "subsystem" available to researchers. At the very least, animal research will become largely obsolete.
Even more exciting is the very real probability of "patient on a chip" models. This will allow clinicians to adjust treatments not just to the general population, but develop laser-focused approaches to individuals cheaply, quickly and effectively.
PCR Lab-On-A-Chip
The PCR test (polymerase chain reaction) is regarded as a gold standard when it comes to detecting the genetic material or viruses. It is contrasted to the somewhat less reliable antigen test, which detects antigens to the specific virus.
A key part of PCR testing is gene amplification, that is, making more copies of the same genetic sequence. This is vital because it makes testing possible with a much smaller initial sample.
As described by Technology Networks[7], PCR performed via microfluidics offers a number of advantages aside from the low sample size concentrations required. These include great sensitivity and speed, which is greatly valued in point of care testing.
Challenges and Opportunities
Rapid diagnosis of disease plays a critical role in containing the spread of infectious diseases. As it stands today, medical diagnostics involves visits to medical facilities where tests can be performed. A much better solution would be for individuals to be able to self-test at home. Microfluidic-based labs-on-a-chip are beginning to make this possible.
Barriers to this solution include the still high price of the chips, but there is every reason to believe that economies of scale will bring costs down. Even then, the necessarily conservative attitude of regulatory authorities will tend to slow down adaptation.
Participants in the field need to realize that for this technology to reach its practical potential, they must begin to look past their laboratories and research centers. There is a need to establish an ongoing dialog with other important stakeholders including regulators, practicing physicians, hospitals and perhaps most importantly, major sources of funding. Collective action is needed.
Wrapping Up
Like computer chips, microfluidic labs-on-a often depend on photolithography in their construction. Instead of electrons flowing through tiny copper vias, liquid to be analyzed or modified flow through microscopic channels. Digital operations are replaced by minuscule mechanical valves, mixers and pumps.
These devices have many use cases, including food safety, drug development, disease detection and environmental safety. Their key value propositions include speed and accuracy. Perhaps of even greater importance is that they are tiny, portable, and can achieve results right on the spot, with little need for access to centrally located laboratory facilities.
References
- What is Microfluidics? Ossila
- An Introduction to Microfluidics Applications: Lab-on-a-Chip to Wearable Sensors. Aline
- Microfluidic Sensors for Point-of-Care Diagnostics. AZO Sensors
- Understanding Flow Sensors: For Microfluidic Syringe and Pressure Pumps. We are Cellix
- Emerging Trends in Microfluidics. Elveflow
- The Rise of the Lab-on-a-Chip. Biotium
- An Introduction to PCR. Technology Networks
Glossary of Terms
- Gene Amplification. Making more copies of a gene or a DNA sequence
- Micro Electro Mechanical Systems (MEMS). A methodology employed in photolithography.
- PCR test (polymerase chain reaction). The gold standard when it comes to detecting the genetic material or viruses. Microfluidic Labs-On-A-Chip are now exploiting this pivotal technology.
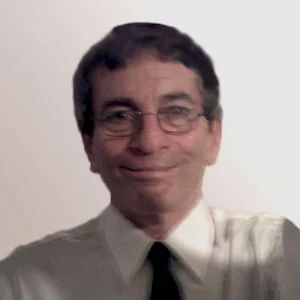