Could Silicon Anodes Be Made More Stable?
21-01-2020 | By Liam Critchley
Batteries are vital components in many modern-day technologies, and for many existing technologies, improving the capabilities of the battery is paramount for building the next generation and improving the status quo.
Li-ion batteries remain the common (rechargeable) battery in use today, and this is down them being the most stable. But even though they are the first choice, others are close to becoming a commercial reality, so Li-ion battery research needs just as much effort for them to remain as the go-to battery.
The key thing with most battery research is that it is not very often that ground-breaking research emerges, rather, it is often a series of incremental advances that leads to a much better system in the long run.
The Li-ion Battery Anode
Li-ion batteries have three vital components that enable them to function – the anode, the cathode and the electrolyte – and a lot of research and development is being invested in all these areas to build better and smaller batteries. The anode is the electrode which discharges the ions (after they have been charged and stored) and loses an electron to generate a current in the process. It also stores the ions during the charging phase.
The anode is always made of conducting material, and for many Li-ion batteries, graphite is the standard material of choice. In recent years, other carbon nanoforms – such as graphene and carbon nanotubes – have been trialled but are not yet a viable large-scale option.
What About Silicon?
Silicon is a material that is used in many electronic devices because it's conductive, can be made in very small architectures, and is relatively easy and cheap to obtain and manufacture. So, many would think that silicon would be an ideal anode material, as it should be safe given that it used in many electronics.
In an ideal world, that would be a yes. However, science throws up many challenges and one has been the realisation of a stable silicon anode in batteries. While silicon is conductive and is ideal from that perspective, the shuttling of ions in and out of the electrode causes the silicon anode to expand and contract, displacing silicon particles and destabilising the solid electrolyte interphase (SEI) layer in the process.
These induced volume changes within the anode ultimately cause it to structurally fail much quicker than other anodes, so their use in commercial batteries has not been an option due to its lack of long-term stability and safety.
New Research Into Silicon Anodes
Given the challenges associated with silicon anodes, research is not generally the quickest or the most fruitful compared to other areas. But recent research published in Nature Communications by Wang et al involves the development of a very interesting electrolyte that stabilises the anode.
The research is interesting from the point that they have focused on stabilising the anode via the electrolyte, whereas most efforts have looked to hybridise the silicon with other materials to build a more physically stable electrode or have looked to coat the electrode to minimise volume changes.
The researchers from China and the USA used a silicon monoxide anode in their battery setup alongside a lithium-based cathode known as an NCM – with a chemical formula of LiNi0.5Co0.2Mn0.3O2. The electrolyte that brought this battery together was a gel-based polymer electrolyte.
The polymer electrolyte was a type of co-polymer, i.e. a polymer which is composed of two different monomer units (the units which make up the chemical backbone of the polymer). The two chosen polymer units were poly(tetramethylene ether) glycol (PTMG) and 4,4’-methylenediphenyl diisocyanate)-ethylenediamine (MDI-EDA). The combination of a soft and a hard polymer created a gel-like substance, and it is the elastic properties of the electrolyte which are the key drivers for the anode being stable.
The excellent elasticity of the gel polymer electrolyte (GPE) arises from both its constituents. The softer PTMG polymer swells in carbonate electrolytes, whereas the harder MDI-EDA polymer prevents the whole copolymer from experiencing excess swelling. The result is a gel electrolyte that has a very high elasticity (over 900% elongation) that can act as a ‘cushion’ for the silicon anode (and as a cushion between the two electrodes).
The gel polymer electrolyte acts as a cushion to stabilise silicon electrode structures.
Credit: Donghai Wang
Given that a conventional silicon anode was chosen (not a specially designed one) for the battery, it would still swell under normal conditions. However, the researchers found that when the electrode swelled, the GPE cushion around the anode significantly reduced the degree of expansion, while simultaneously reducing the number of silicon particles displaced from the anode. Over time, this also reduced the amount of cracking that these anodes normally experience and simply made the basic silicon anode have a longer usable life.
While the electrolyte is not perfect, it marks a significant improvement in the usability of basic silicon anodes and this GPE can now act as means for newer and more efficient GPEs to be synthesised and trialled. Even this electrolyte had a capacity retention of 70% over 350 cycles, alongside a 99.9% Coulombic efficiency, and it’s more than likely that any future electrolytes which use similar methods of anode protection will be even more effective.
Future Outlook
Silicon-based electrodes are still a long way from being used in a commercial setting, but out-of-the-box thinking towards finding solutions for the issues of volume expansion and anode instability could be key for future developments. It’s unlikely that this specific electrolyte will be used commercially (although you never know), but research of this capacity is certainly able to inspire more advanced research in the future. Whether we eventually see silicon anodes in commercial products, no-one has the answer, but the advances in the technology are certainly further ahead than they have been in recent years.
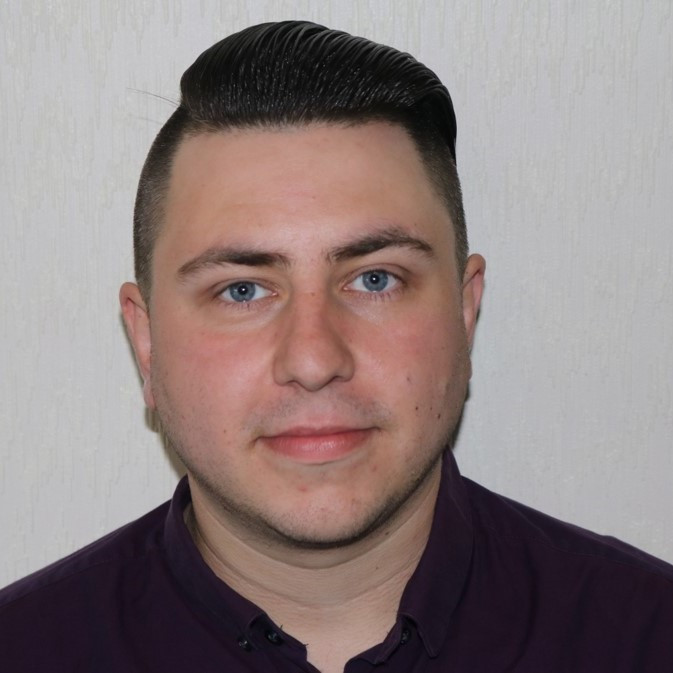