Surface Engineering of Perovskite Solar Cells
30-09-2020 | By Liam Critchley
Different types of solar cells can be tailored and optimised to be more efficient. This is often due to surface engineering approaches maximising the performance of the active regions of a solar cell. Out of all the different (inorganic) solar cells being researched and manufactured, perovskite solar cells show some of the highest efficiencies and some of the biggest potential for large scale photovoltaic adoption. However, several different issues have currently held them back from being a large-scale commercial success, so far.
Perovskite materials are a type of inorganic material with a chemical structure of A2+B4+X32—where A and B are positively charged cations and X is a negatively charged anion. Of the many different perovskites in existence, lead-halide perovskite solar cells have shown some of the highest efficiencies within the class of pure inorganic solar cells (the category with the highest efficiencies). They are typically of low cost to produce due to being solution processable.
In recent years, the focus of research has shifted, and a lot of efforts are now centred around creating solar cells with hybrid perovskite materials—which are part inorganic and part organic in nature. However, these devices have their issues as well, so there are also other research efforts going into creating all-inorganic lead-based perovskite materials (using other metals as well) that function better than the pure lead-based perovskite materials commonly trialled for solar cells.
All-inorganic Lead-Based Perovskite Solar Cells
While hybrid perovskite cells can be made up of many different materials in a range of combinations, many of the lead hybrid perovskite materials degrade under heat because the cations used are volatile. This makes a lot of them unusable for solar cell applications, and one of the solutions to combat this over the years has been to replace the volatile organic cations within hybrid lead perovskite materials with caesium ions to create all-inorganic perovskite materials.
By replacing the volatile organic cations with more stable inorganic cations, the resulting perovskite materials are more stable under high temperatures and often have a tunable bandgap. These all-inorganic perovskites can utilise several anions within the structure, but so far, CsPbI2Br has shown some of the higher power conversion efficiencies. However, these devices are still far from perfect, and there are still some issues that need to be ironed out if their real-world potential is to be realised.
Issues with Current Caesium-Lead Perovskite Solar Cells
One of the main issues of caesium-lead perovskite solar cells is that even though they are more stable under high temperatures than organic-inorganic structures, they have their instabilities—which tend to be light and moisture instabilities. These instabilities have been pinpointed to the internal defects within the inorganic lattice structure of the perovskite, typically from grain boundaries or where the internal surfaces have been enriched with uncoordinated or mobile ions.
The defects present within the surfaces of many caesium-lead perovskite materials also induce trap states which cause a process known as nonradiative charge recombination—which when a phonon is released instead of a photon when the charge carriers (electrons and holes) recombine at the semiconducting junction. These processes are unfavourable and can lead to a reduction in device efficiency.
Engineering the Surface of Caesium-Lead Perovskite Solar Cells
One of the main ways to combat issues with surface defects is to alter the surface layer itself or coat it so that its properties change. This is a process known as surface passivation and has been used to improve the performance of many other solar cells, as well as the properties of many other materials and devices not related to solar cells. These methods act as a way of engineering and tailoring the surface of materials so that they function better, are more stable, or possess fewer defects.
There have been several different molecules which have been used to passivate the surface of photoactive perovskite materials to reduce the defects, better align the intrinsic energy levels of the materials, increase the stability of the device, and minimise ion migration within the photoactive junction. One of the key ways that many surface passivation/engineering approaches have been successful is through the formation of a strong chemical bond with the passivating agent—as it makes it harder to remove via the environmental stimuli, such as moisture or temperature. So, a lot of research is looking into these devices to see how they can engineer the surface chemically rather than physically.
A research team from China and Australia has recently employed surface engineering methods to CsPbI2Br perovskites in an attempt to negate the effects of the inherent defects within the material. The team used a chemical binding molecule known as diethyldithiocarbamate (DDTC) which bound strongly to and anchored, the surface lead cations, remaining thereafter the chemical process was over and when the device was active. This passivation process removed the negative effects of any uncoordinated lead ions and iodine/bromine defects within the perovskite and improved the properties of the perovskite in the process.
Engineering the surface of the perovskites in this way led to an overall improvement in device performance across many key areas. This included a high power conversion efficiency of 17.02% and a very high stability to both light irradiation and moisture—negating two of the key issues with many of these perovskite solar cells—as well as an ability to maintain efficiency of over long operational periods.
The high stability and performance properties have been attributed to the strong and stable bonding of the DDTC to the surface of the perovskite. There were several other organic molecules which were found to work well with the lead ions, but DDTC worked best. This means that many potential solutions are going forward that can be trialled commercially, and it’s possible that the other stabilising agents could be useful for other active solar cell materials.
Conclusion
While the surface engineering methods employed recently have been used to optimise the performance of lead-based perovskite solar cells, it is a process that is not limited to these specific devices and materials. Surface engineering has offered a way to optimise many materials and solar cells, as well as other technologies. As surface engineering techniques advance, we will likely find similar processes being implemented to improve a wide range of technologies.
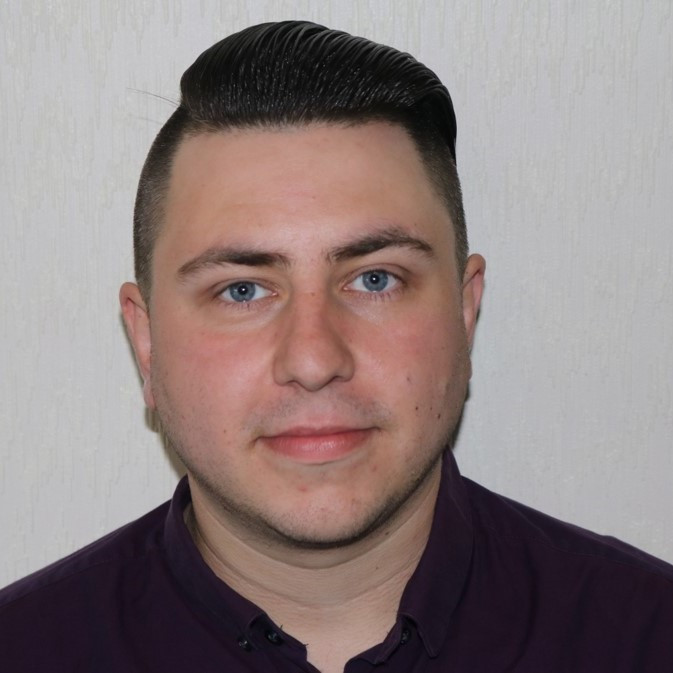