Removing Defects in Perovskite Photovoltaics with Dielectric Screening
24-05-2021 | By Liam Critchley
Solar cells are one of the most promising options for obtaining large amounts of energy (on a global level) by renewable means. There are many types of solar cells (otherwise known as photovoltaics) out there which use different materials, all of which have different power conversion efficiencies (PCEs) and are suitable for different environments. The efficiencies of all types of solar cells have been increasing year on year and we’re now starting to see solar cells being rolled out on mass levels.
Given the need for renewable energy in a modern-day, greener society, scientists are still trying to make solar cells more efficient so that we can obtain more energy per area in large solar farms or reduce the number of solar cells in a given area (by improving the efficiencies of each device so that fewer cells can harvest just as much energy as now). One of the most promising candidates from a purely PCE perspective is perovskite solar cells.
Perovskite Photovoltaics
All-inorganic solar cells—where the semiconducting junction responsible for turning sunlight into an electrical current is an inorganic material—have higher efficiencies than other materials, but they are less flexible so are only used in more traditional settings (i.e., not on walls like organic solar cells). Perovskites are one such example, and an efficient example at that.
A perovskite material is material with a very specific structure classified by having certain ions (and relative charges of these ions) in its structure. All perovskites—of which there are a lot—have a chemical structure of A2+B4+X32-, where the A and the B refers to two different cations (with a 2+ and 4 + charge, respectively) and are linked together by an anion, which is termed ‘X’.
Perovskite materials can absorb wavelengths of light from across the whole electromagnetic spectrum. In addition to this, they also possess long charge carrier distances, the ability to be tuned easily, and the active charge carriers have a rapid charge separation. This combination of properties is responsible for their high PCEs, and they are also responsible for a lot of interest being shown in them for photovoltaic devices.
The Push to Remove Defects
Despite already showing high PCEs and possessing excellent wavelength absorption properties, the current day photovoltaic cell efficiency is still lower than the theoretical limit—which is defined by the Shockley-Queisser theory that calculates the potential theoretical efficiency of a solar cell (with a single p-n junction), where the only loss mechanism is radiative recombination in the solar cell. So, to get close to the theoretical limit, the nonradiative losses need to be mitigated.
It is thought that the PCE of different perovskite solar cells could become closer to the theoretical limit in the near future if scientists can identify and remove defects from the junction by better understanding the mechanisms of electron or hole capture by the defects. Knowing these parameters will enable solar cell manufacturers (and fundamental scientists) to develop effective strategies for suppressing any of these undesirable energy loss pathways in and around the p-n junction of the solar cell.
Defects in solar cells are detrimental to the performance of the device (like they are in almost any application). The presence of defects in the inorganic lattice of the p-n junction can increase the scattering of charge carriers during the transport process, which leads to altered phonon energies and a change in electron-phonon coupling strength. In perovskite solar cells, defects have already been proven to be the reason for ion migration and device instability, so removing them should help to improve their long-term usability.
A Dielectric Screening Approach
A number of strategies have already been employed to improve the PCE of perovskite solar cells. However, many of them have failed to provide an in-depth understanding of how electrons and holes interact with defects. In many traditional semiconductor junctions, it is thought that the Coulomb attraction between the defects and charge carriers increases the defect capture cross-section, leading to more defects being captured. It has also been stated previously that the charge carriers in some perovskites could be protected from scattering if they behave as polarons (a quasi-particle).
There have been some efforts previously that have been able to screen the intrinsic defects and manage the effects internally. But a lot of efforts to date have not considered the external engineering effects that could be utilized and be used to manipulate the intrinsic defect-carrier interactions, and therefore improve device performance.
A new study has looked at how external factors can be used to improve the PCE of perovskite devices, as well as provide insights into how defects and charge carrier interactions contribute to lower PCEs, enabling a picture to be built up about how screening approaches can affect device performance. The approach used was a dielectric screening effect, which was enabled by controlling the space charge within perovskites. The study found that controlling dielectric response is a feasible pathway to achieve screening in these solar cells.
In this study, the team found that the dielectric constant is a bridge connecting the Coulomb interaction of defects and phonons with charge carriers. Changes in the dielectric response of the perovskites led to a large variation of Coulomb interactions, and therefore provided a dielectric screening effect. The dielectric screening effect lowered the possibility that charge carriers may become trapped in defects. Even in parts of the material where defects remained, they became ‘invisible’ to the charge carriers, as if they were in some type of ‘stealth mode’.
Therefore, it was also found that the potassium halide species in the perovskite chosen could reduce defect carrier capture cross-sections at the grain boundaries and suppress electron-phonon coupling in the junctions. These interactions effectively screened the carrier nonradiative pathways. The perovskites screened in this manner also showed reduced surface recombination, so a number of factors contributed to the overall performance of the device and boosted the PCE of the device up to 22.3% with an open-circuit voltage of 1.25 V and a low voltage deficit of 0.37 V.
The work done is this study was not only able to provide and an in-depth understanding of the carrier capture processes in perovskites, but it also enabled the researchers to quantify the specific voltage losses from the film to the full device (by measuring the effects of defects from different angles), providing insights into the main voltage loss pathways in perovskite solar cells. Aside from generating insights, the work provided a promising route towards realising highly efficient perovskite devices via dielectric regulation. If we are to see a wider use of perovskite solar cells (and all solar cells), then understanding and mitigating the effects of defects in critical areas of the device is going to be very important going forward.
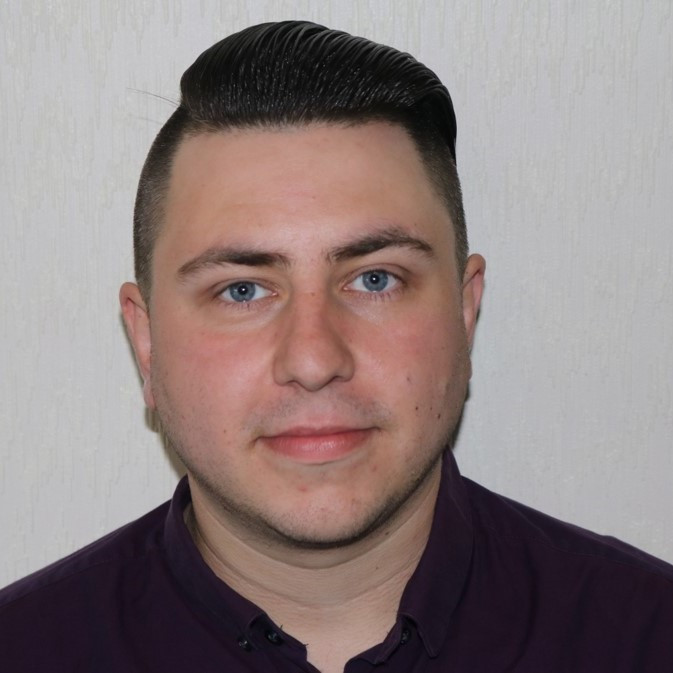