Potassium-Sulphur Batteries: Optimisation Leads New Energy Era
06-03-2024 | By Liam Critchley
Key things to know:
- Potassium-Sulfur Batteries (KSBs) offer a high theoretical energy density of 1023 Wh kg-1, leveraging the abundant and cost-effective resources of potassium and sulfur.
- Challenges such as low sulfur utilisation and sluggish kinetics of potassium polysulfide conversion need to be addressed to harness the full potential of KSBs.
- Innovative approaches like the tungsten-carbon composite have been developed to improve the conversion of potassium-sulfur intermediates and mitigate issues like catalytic poisoning.
- Metal-Organic Frameworks (MOFs) are being explored to create functional hosts that can efficiently manage the sulfur ions, enhancing the stability and performance of KSBs.
In the quest for sustainable and efficient energy storage solutions, the exploration of alternative battery technologies has become paramount. Among these, Potassium-Sulfur Batteries (KSBs) emerge as a promising contender, offering a blend of high energy density and cost-effectiveness derived from the abundant resources of potassium and sulfur. This article delves into the innovative approaches and recent advancements in KSB technology, particularly focusing on the challenges and solutions related to stability and performance, thereby paving the way for a potential shift in the landscape of energy storage systems.
Introduction to Advanced Battery Technologies
If you look beyond the veil of Li-ion commercialisation, there are a number of battery architectures out there which have a lot of potential due to having a higher theoretical energy density than Li-ion batteries. However, for one reason or another, many of these batteries have never seen the light of day in our consumer technologies due to varying levels of long-term instability and device degradation.
As the demand for higher power and more efficient energy storage systems becomes greater, there is more interest being generated in battery architectures beyond the conventional Li-ion battery. One of these is potassium-sulphur batteries (KSBs).
Recent advancements in KSB technology have highlighted the potential of tungsten-based catalytic composites in optimising potassium polysulfides, a crucial aspect for enhancing KSB electrochemistry. This innovative approach aims to address some of the inherent challenges in KSBs, such as the low sulfur utilisation and the sluggish kinetics of potassium polysulfide conversion, by facilitating a more efficient redox process within the battery. Read more about this research in Nature Communications.
KSBs have a high theoretical energy density due to the high capacity of the sulphur redox reaction that occurs within the battery and has been touted to have a theoretical energy density of 1023 Wh kg-1. One of the other benefits of KSBs is that they are low cost due to the natural abundance of potassium and sulphur in the Earth—and the availability of materials is something that has been thrown around a lot recently as a cause for concern with the scale-up of EV batteries and the mining of finite metals for battery systems.
Challenges to Overcome: Stability Issues of KSBs
However, like many non-commercial battery systems, there are still a number of inherent stability issues that need to be overcome before KSBs even get a sniff of becoming a consumer battery system. A lot of the challenges with KSBs revolve around the sulphur cathode, as it is a detrimental factor during normal operation.
For example, the sulphur cathode typically has a lower sulphur utilisation, and these systems often have slow and sluggish kinetics towards potassium polysulphide conversion. There are also issues with the shuttling of ions (something that all sulphur batteries exhibit; just look at the issues with lithium-sulphur batteries over the years) and the decomposition of potassium-sulphur compounds in the battery. As the ions escape from the pores of the cathode and form long molecular chain intermediate species, they block the pores of the cathode (where the ions would go in and out during cycling), causing poor chemical performance and fast failure rates of the electrochemical cell.
Like other sulphur-based batteries, there are challenges with intermediates forming due to the shuttling of sulphur ions. These challenges also limit the capacity and lifespan of KSBs, like they have in other batteries, so finding ways of containing the sulphur ions so that they don’t shuttle and form intermediates that affect the electrochemical performance and cell stability is key. This has been taking the form of using functional hosts to contain the sulphur ions.
Carbon-based Materials are the Common Host Material
Different functional hosts for KSBs have been attempted over the years, but the majority of these have been carbon-based materials due to the good electrical conductivity, diverse surface chemistry, and versatile porosity that many carbon materials offer. In these approaches, confining the sulphur atoms has been the key driver and has been achieved by physically containing the sulphur atoms within the carbon network of the host. However, many of these carbon networks have had to be doped with nitrogen atoms to improve the sulphur loading due to limitations in pore volume, but the doping of these materials led to sulphur atoms being more tightly anchored within the carbon network. The use of some carbon materials, such as metal carbides, as well as other single metal atoms, has previously been reported to have good adsorption effects towards polysulphide molecules.
In the pursuit of optimising potassium-sulfur batteries (KSBs), researchers have employed advanced theoretical models and computational screening techniques. The following figure, "Theoretical guidance and screening for sulfur host design," encapsulates the rigorous process undertaken to identify the most promising materials and configurations. This strategic approach is pivotal in addressing the inherent challenges of KSBs, aiming to enhance their efficiency and stability through innovative sulfur host designs.
a The affinity measurements, represented as binding energies, for K2S4 across various substrates including WSA@NC, W metal (110), W2C (102), WC (101), WN (112), WO3 (100), WS2 (002), and WSe2 (006), along with their respective structural layouts (shown in insets). b The energetic pathways for K2S2's movement across NC, WSA@NC, and W2C (102) surfaces. c The delineated stages - initial, intermediary, and concluding - of K2S2's journey. d A graphical representation, known as a volcano plot, illustrating the relationship between the energy metrics and the dual processes of KPS conversion and migration. e A diagram outlining the conceptual framework behind the WSA-W2C hybrid as a sulfur host, aimed at optimising sulfur redox reactions within KSBs, as inferred from theoretical analyses. The foundational data for these insights are accessible in the Source Data file.
Tungsten-Carbon Approach Developed to Tackle Challenges
Previous efforts to contain the sulphur ions have revealed that introducing catalytically active sites into the host material improves the conversion of potassium-sulphur intermediates back into their original ion states. However, potassium-sulphur electrochemistry is a complex area, and researchers believe that more factors should be considered beyond catalytic conversion alone. One of the issues that they believed had been overlooked is the possibility of catalytic poisoning—as some of the reduction products are insulating in nature and difficult to decompose, so they could accumulate over catalytic sites, causing catalytic poisoning (deactivation of the catalytic area).
The introduction of tungsten single atom and tungsten carbide within a nitrogen-doped carbon framework has been identified as a promising strategy to enhance the migration and conversion of potassium polysulfides. This dual-functional approach not only catalyses the conversion process but also prevents the accumulation of inert reduction products, thereby mitigating the risk of catalytic poisoning. Such advancements underscore the importance of material innovation in overcoming the stability issues of KSBs. Further details can be found in the original study.
It's thought that migrating the atoms once they have been decomposed at the catalytic site could prevent the build-up of inert atoms. This approach was thought to help to prevent the expansion of electronically inactive areas of the cathode. As this area has been previously overlooked, researchers looked towards creating a sulphur host that not only broke down the intermediate species but also removed the resulting reduction products away from the active catalytic sites.
Innovative Solutions: Metal-Organic Frameworks (MOFs)
Researchers have taken to using a metal-organic framework (MOF)—a porous hybrid material that uses metal ion complexes to define the size and shape of the pores, combined with organic linker and ligand molecules that form the rest of the porous network and are used to interact with hosts inside the pores.
The researchers created two different ligand environments within the MOF to attach and functionalise the pores with tungsten-based groups—to create the functional aspects for intermediate breakdown and removal. On the one hand, tungsten carbide groups within the pores were used as the catalytic sites for breaking down the potassium polysulphide chains into short-chain K2S and K2S2 chains. These are then accelerated away from the catalytic site by the tungsten atoms due to lower migration energy barriers. This avoids the build-up of K2S at the active catalytic site, avoids catalytic poisoning, and allows for the consecutive decomposition of K2S species.
The synergy between tungsten carbide and single tungsten atoms, as revealed through density functional theory (DFT) calculations, plays a pivotal role in the enhanced performance of KSBs. This combination not only facilitates the efficient breakdown of potassium polysulfide chains but also ensures the rapid migration of the resulting short-chain species away from the catalytic sites, thereby enhancing the battery's overall efficiency and longevity. Discover more about the impact of this research on KSB performance.
The choice of using tungsten carbide and single tungsten atoms was determined by density functional theory (DFT) calculations, as these studies not only showed the catalytic and migration ability but also an enhanced cycling rate and performance.
From Theory to Practice: Realising MOF-Enhanced Batteries
When put into practice, the battery was created using the MOF hosts, enabling a highly efficient potassium-sulphur battery to be created. The technical aspects of the battery included a sulphur utilisation of 89.8%, a capacity of 1504 mAh g-1, a rate capability of 1059 mAh g-1 at 1675 mA g-1 and a testing cycling stability of 200 charge cycles (which is high but not to commercial Li-ion standards).
Naturally, the number of cycles that the battery is capable of performing under is something that would inevitably have to be improved on over time to be commercially feasible—as many modern Li-ion systems are now usable over 1000 cycles—but the focus in the research was on preventing the potassium-sulphur intermediate molecules from affecting the electrochemical performance of the battery.
To that end, the research has been successful, and it was similar to the types of research that were fundamentally needed to overcome the polysulphide shuttling charges of lithium-sulphur batteries. Lithium-sulphur batteries are now being commercialised after years of facing these types of challenges, so if research continues into potassium-sulphur batteries, then they could have the potential to be commercialised in the future as well.
The practical application of these tungsten-based catalytic composites in KSBs has demonstrated significant improvements in sulfur utilisation, capacity, rate capability, and cycling stability. These results not only contribute to the body of knowledge in battery technology but also pave the way for future research and development efforts aimed at commercialising KSBs as a viable alternative to traditional Li-ion batteries. The continuous exploration of such innovative solutions is crucial for advancing the field of energy storage systems. Access the full research article for comprehensive insights.
Reference:
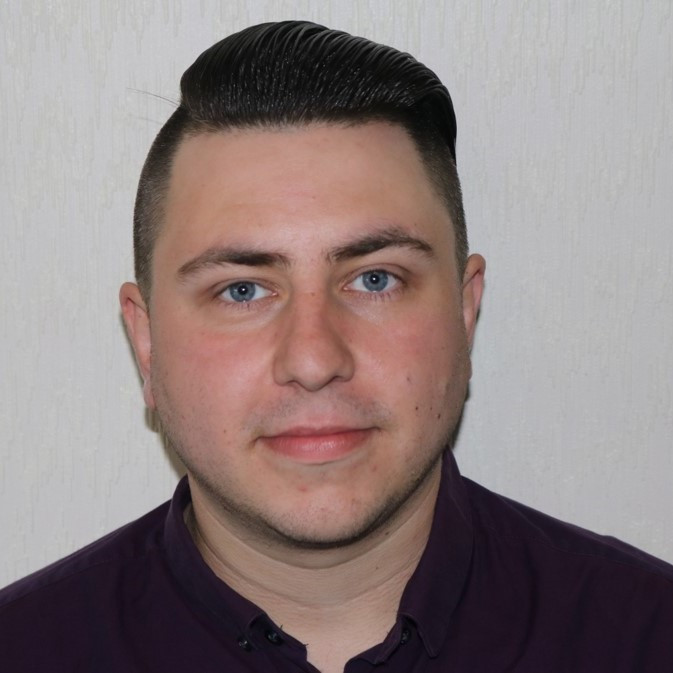